COVID-19 treatment: systemic agents
• Many systemic agents reduce risk
@CovidAnalysis, April 15, 2025
We do not provide medical advice. No treatment is
100% effective, and all may have side effects. Protocols combine multiple
treatments. Consult a qualified physician for personalized
risk/benefit analysis.
Over 8,000
compounds predicted to reduce COVID-19 risk. SARS-CoV-2 is easily
disabled.
SARS-CoV-2 infection and replication involves a complex
interplay of over 100 host and viral proteins and other
factors1-8, providing many therapeutic
targets.
Scientists have identified 8,959+
compounds9 potentially beneficial
for COVID-19. Hundreds of compounds inhibit SARS-CoV-2 in vitro,
including many with established pharmacokinetic profiles and proven safety.
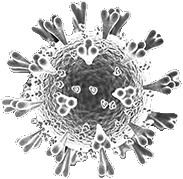
c19early.org
Efficacy confidence - Low-cost systemic agents | ||
Hydroxychloroquine | p<0.0000000001 | |
Ivermectin | p<0.0000000001 | |
Metformin | p<0.0000000001 | |
Curcumin | p=0.00000001 | |
Antiandrogens | p=0.00000006 | |
Colchicine | p=0.0000002 | |
Melatonin | p=0.0000002 | |
Probiotics | p=0.000001 | |
Azvudine | p=0.000002 | |
N-acetylcysteine | p=0.00003 | |
Antihistamine H1RAs | p=0.00007 | |
Fluvoxamine | p=0.0001 | |
Nigella Sativa | p=0.0002 | |
Famotidine | p=0.0003 | |
Quercetin | p=0.002 | |
Efficacy confirmed March 2020 (HCQ)(a),10 |
Hydroxychloroquine.
Most HCQ studies (90%) focused on late treatment. Early/preventive
trials show selective reporting—failing to report results at 3x the rate of
late treatment studies, suggesting suppresion of positive results.
11 In Silico986-996, 24 In Vitro986,997-1019, and 3 animal1001,1011,1020 studies support the efficacy of hydroxychloroquine.
Viruses like SARS-CoV-2 that depend on low pH for endosomal
entry1021,1022 can be inhibited with endosomal
acidification inhibitors like
HCQ(c),998,1011,1022.
Direct clinical measurement shows that HCQ reaches therapeutic concentrations
in COVID-19 patients1023, and analysis of lung cells from COVID-19
patients shows inhibition in early target cell types1024.
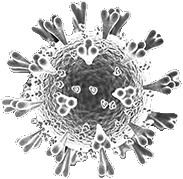
Analysis of 423 controlled
clinical studies117-542 shows very
strong evidence for efficacy with early treatment
(p<0.0000000001,
38 studies) and
prophylaxis (p<0.0000000001,
118
studies), confirmed in multiple additional meta analyses418,1025-1032.
The largest HCQ/CQ RCT—the 4,652 patient Oxford/MORU COPCOV
RCT—shows 57% lower symptomatic PCR+ COVID-19 with
HCQ/CQ (p = 0.0002)(b),533.
In 2021 Naggie et al.485 showed that HCQ pre-exposure prophylaxis
significantly reduces COVID-19 cases based on 2 US RCTs. This is now known with
p=0.00004 from RCTs and
p=0.00000001 for observational studies.
HCQ shows poor results with late treatment and excessive dosage, and the
combination shows harm.
Late-stage HCQ treatment may enhance viral egress via lysosomal
deacidification(d),1033,1034. Research also suggests potential
cardioprotective effects at lower doses, but cardiotoxicity with excessive
dosage1002.
Studies for HCQ are inconsistent with the logical use of
antivirals. 90% of treatment studies have analyzed late treatment,
5+ days after the onset of symptoms543.
This makes it easy to generate meta analyses showing poor efficacy by
including large late treatment studies1035, although the results
are not relevant for recommended usage.
Results have not been reported for 37 RCTs1036-1072, and an
additional 65 HCQ RCTs were terminated with <30
patients1073-1131.
HCQ was the first treatment confirmed
effective1132, however alternatives
may offer advantages. Lung pharmacokinetics show high inter-individual
variability1023; dosage is relatively challenging, with dependence
on cholesterol1005, lung pH996, and renal
impairment996, delayed attainment of therapeutic
concentrations, and a relatively narrow range of regimens showing efficacy
while limiting side effects; and ~2.5%1133 of patients may have
contraindications.
Longer-term use of endosomal acidification modifiers for prophylaxis raises
concern for potential off-target effects, including disruption of cellular
processes, impaired lysosomal function, reduced immune
response1134, and altered cellular signaling.
Fake tablets are common in some locations1135,1136.
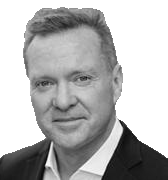
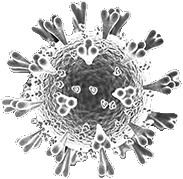
❝
There is a clear signal that IVM works in COVID patients ...
Ed Mills, Together Trial co-principal
investigator1137
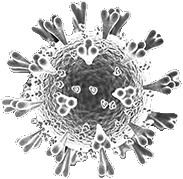
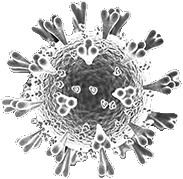
Analysis of 105 clinical
studies11-115 shows very strong
evidence for efficacy (p<0.0000000001), confirmed in multiple additional meta analyses1227-1233.
Ivermectin shows efficacy in RCTs for prophylaxis,
early treatment, and late treatment.
The major RCTs claiming no benefit actually show positive results, despite
extreme bias1234.
The largest trial, with a design highly favoring
finding no efficacy, found superiority with probability > 0.999 for
recovery96. Improved recovery is very strongly associated
with lower mortality, p<0.0000000000196. The trial also
found 36% lower long COVID (p<0.0001) despite very suboptimal
use96.
Ivermectin also has the most carefully analyzed evidence base
in history, resulting in the retraction of a few studies which has improved
the quality of the evidence base, and improved the dose-response and treatment
delay-response relationships. However the analysis team focused only on issues
with positive
results1235,
showing very strong
bias1236,1237
and disregarding all flaws and fraud in the major trials
claiming no effect116.
For example, the main author claims the Together Trial was "incredibly
well-done" and a "masterpiece of science" despite the trial
reporting multiple impossible numbers, blinding being broken with external
sharing during the trial, randomization failure, refusal to share data despite
pledging to, no response from the authors, and many protocol violations.
Optimal use of ivermectin may involve synergy with combined treatments,
administration taking into account the lipophilic nature, and sublingual,
spray, or inhaled formulations for direct treatment to the respiratory tract.
Pharmacokinetics show significant inter-individual variability1238.
Injectable formulations may reduce variability and provide much faster onset
of action1188.
Liposomal formulations show increased antiviral activity and lower
cytotoxicity1181.
Synergistic results are seen with polytherapy1178,1180,1186.
Efficacy varies depending on the manufacturer1239, underdosed
and contaminated ivermectin is common1240-1243,
and fake tablets with no active ingredient have been
reported1244.
Metformin.
4 In Silico1143,1245-1247, 7 In Vitro551,627,1246,1248-1251, and 4 animal627,1248,1249,1252 studies support the efficacy of metformin.
A systematic review and meta-analysis of 15 non-COVID-19 preclinical studies showed that metformin inhibits pulmonary inflammation and oxidative stress, minimizes lung injury, and improves survival in animal models of acute respiratory distress syndrome (ARDS) or acute lung injury (ALI)1253.
Metformin inhibits SARS-CoV-2 in vitro551,1251, minimizes LPS-induced cytokine storm in a mouse model1252, minimizes lung damage and fibrosis in a mouse model of LPS-induced ARDS627, may protect against SARS-CoV-2-induced neurological disorders1250, may be beneficial via inhibitory effects on ORF3a-mediated inflammasome activation1254, reduces UUO and FAN-induced kidney fibrosis627, increases mitochondrial function and decreases TGF-β-induced fibrosis, apoptosis, and inflammation markers in lung epithelial cells627, may reduce inflammation, oxidative stress, and thrombosis via regulating glucose metabolism1246, attenuates spike protein S1-induced inflammatory response and α-synuclein aggregation1249, may reduce COVID-19 severity and long COVID by inhibiting NETosis via suppression of protein kinase C activation1255, enhances interferon responses and reduces SARS-CoV-2 infection and inflammation in diabetic models by suppressing HIF-1α signaling1248, and may improve outcomes via modulation of immune responses with increased anti-inflammatory T lymphocyte gene expression and via enhanced gut microbiota diversity1256.
104 metformin clinical
studies415,421,544-645 show
efficacy, confirmed in multiple additional meta analyses1257-1278.
Efficacy has also been shown for influenza A1279.
Antiandrogens.
49 antiandrogen clinical
studies647-695 show
efficacy, confirmed in multiple additional meta analyses1280,1281.
Potential mechanisms include inhibition of TMPRSS2 expression, reduction of
androgen-mediated viral entry, modulation of the immune response, and
attenuation of inflammation.
Antihistamine H1RAs.
4 In Silico1282-1285, 7 In Vitro699,707,1283,1285-1288, and 2 animal1286,1289 studies support the efficacy of antihistamine H1RAs.
17 antihistamine H1RA clinical
studies697-713 show
efficacy.
Curcumin.
26 In Silico987,1140,1143,1171,1290-1311, 25 In Vitro999,1290,1291,1294,1296,1300,1305,1309,1312-1328 , and 1 animal1294 studies support the efficacy of curcumin.
In Silico studies predict inhibition of SARS-CoV-2 with curcumin or metabolites via binding to the spike(e),1140,1290,1294,1299,1301,1306,1309 (and specifically the receptor binding domain(f),1297,1300,1303), Mpro(g),1140,1290,1294,1296,1298-1300,1302-1304,1307,1309-1311,1325 , RNA-dependent RNA polymerase(h),1140,1290,1300,1308, PLpro(i),1140, ACE2(j),987,1301,1302, nucleocapsid(k),1171,1295, nsp10(l),1171, and helicase(m),1315 proteins.
In Vitro studies demonstrate inhibition of the spike(e),999 (and specifically the receptor binding domain(f),1328), Mpro(g),999,1305,1325,1327, ACE2(j),1328, and TMPRSS2(n),1328 proteins, and inhibition of spike-ACE2 interaction(o),1313.
Curcumin is predicted to inhibit the interaction between the SARS-CoV-2 spike protein receptor binding domain and the human ACE2 receptor for the delta and omicron variants1297, decreases pro-inflammatory cytokines induced by SARS-CoV-2 in peripheral blood mononuclear cells1324, alleviates SARS-CoV-2 spike protein-induced mitochondrial membrane damage and oxidative stress1291, may limit COVID-19 induced cardiac damage by inhibiting the NF-κB signaling pathway which mediates the profibrotic effects of the SARS-CoV-2 spike protein on cardiac fibroblasts1314, and inhibits SARS-CoV-2 ORF3a ion channel activity, which contributes to viral pathogenicity and cytotoxicity1320.
27 curcumin clinical
studies715-741 show
efficacy, confirmed in multiple additional meta analyses1329-1332.
Bioavailability.
Curcumin has low bioavailability and
stability1333. Extensive research has been done on advanced
formulations which has resulted in >50,000 times improved
bioavailability1334, and efficacy for COVID-19 may depend heavily on
the use of advanced formulations.
Melatonin.
3 In Silico1335-1337, 1 In Vitro1338, and 3 animal1337,1339,1340 studies support the efficacy of melatonin.
Melatonin may restore altered redox homeostasis in COVID-191341, modulates type III interferon responses and reduces inflammatory cytokine production in TLR3 receptor agonist stimulated viral inflammation while preserving tissue integrity1338, and negatively regulates genes critical for viral entry in lung tissue, including reduced expression of FURIN and components of the CD147 complex, while potentially disrupting TMPRSS2/ACE2-mediated entry mechanisms1337.
Melatonin reduces oxidative stress, inhibits NET formation, and protects tissues through anti-inflammatory and antioxidant actions1342.
18 melatonin clinical
studies743-760 show
efficacy, confirmed in multiple additional meta analyses1343-1349.
Potential mechanisms include antioxidant, anti-inflammatory, and
antithrombotic effects, immune modulation, regulation of autophagy, modulation
of the renin-angiotensin system, promotion of sleep and circadian rhythm, and
mitigation of cytokine storm.
Colchicine.
57 colchicine clinical
studies415,762-817 show
efficacy, confirmed in multiple additional meta analyses1350-1359.
Potential mechanisms include direct antiviral activity,
immunomodulatory and anti-inflammatory effects, cardioprotective effects, and
prevention of microvascular thrombosis.
Results are poor with very late treatment780—risks due
to side effects may exceed benefits.
Probiotics.
28 probiotics clinical
studies819-846 show
efficacy, confirmed in multiple additional meta analyses1360,1361.
Efficacy has also been shown for the common cold1362.
NAC.
1 In Silico1143, 8 In Vitro1314,1363-1369, and 1 animal1365 studies support the efficacy of N-acetylcysteine.
N-acetylcysteine shows dose-dependent inhibition of SARS-CoV-21364,1367,1369, shows anti-inflammatory and immunomodulatory effects against SARS-CoV-2-induced immune responses in combination with bromelain1366, suppressed virus-induced reactive oxygen species and blocked viral replication in a humanized mouse model and in human lung cells1365, may limit COVID-19 induced cardiac damage by boosting cellular antioxidant defenses and potentially mitigating the oxidative stress caused by spike protein-induced ROS production in cardiac fibroblasts1314, and reduces disulfide bonds in proteins and exhibits antioxidant properties that may inhibit viral replication and modulate inflammatory responses1363.
NAC may be beneficial for COVID-19 by replenishing glutathione stores and reinforcing the glutathione peroxidase-4 pathway to inhibit ferroptosis, an oxidative stress-induced cell death pathway implicated in COVID-191370.
NAC reinforces glutathione levels, reduces ROS, and minimizes ferroptosis and cytokine storm1342.
24 N-acetylcysteine clinical
studies883-906 show
efficacy, confirmed in another meta analysis1371.
Efficacy has also been shown for influenza1372.
Fluvoxamine.
2 In Silico1373,1374 and 2 In Vitro1374,1375 studies support the efficacy of fluvoxamine.
Fluvoxamine may inhibit SARS-CoV-2 cell entry by preventing the formation of ceramide platforms that facilitates viral uptake1373 and may help restore autophagic processes disrupted by NSP6, thereby reducing SARS-CoV-2 replication and improving host cellular defenses1375.
21 fluvoxamine clinical
studies908-928 show
efficacy, confirmed in multiple additional meta analyses1376-1383.
Potential mechanisms include ASM inhibition, σ-1 receptor activation,
antiplatelet effects, endolysosomal interference, HO-1 increase, reduced cytokine
storm, and elevated melatonin.
Azvudine.
1 In Silico1384, 1 In Vitro1384, and 1 animal1384 studies support the efficacy of azvudine.
34 azvudine clinical
studies848-881 show
efficacy, confirmed in multiple additional meta analyses1385-1388.
Nigella Sativa.
15 In Silico987,1389-1402, 5 In Vitro1393,1395,1403-1405, and 2 animal1400,1406 studies support the efficacy of nigella sativa.
14 nigella sativa clinical
studies930-943 show
efficacy, confirmed in multiple additional meta analyses1407,1408.
Potential mechanisms include direct antiviral activity, and immunomodulatory,
antioxidant, and anti-inflammatory action.
Famotidine.
30 famotidine clinical
studies410,415,945-971 show
efficacy.
Potential mechanisms include direct antiviral activity,
anti-inflammatory effects and reduced cytokine release, reduced acidity
altering conditions for viral replication, immune modulation, and
anticoagulant effects.
Quercetin.
47 In Silico987,989,1140,1290,1299,1302,1311,1409-1448 , 25 In Vitro1290,1313,1320,1325,1409-1411,1413,1423,1427,1429,1432,1446,1449-1460 , and 6 animal1413,1427,1455,1457,1461,1462 studies support the efficacy of quercetin.
In Silico studies predict inhibition of SARS-CoV-2, or minimization of side effects, with quercetin or metabolites via binding to the spike(e),989,1140,1290,1299,1302,1417,1418,1430,1432,1435,1443,1445,1446,1463 , Mpro(g),989,1140,1290,1299,1302,1311,1415,1417,1419,1421,1423,1425,1426,1428,1431,1435,1439,1441,1442,1446-1448 , RNA-dependent RNA polymerase(h),1140,1290,1411,1417,1437 , PLpro(i),1140,1442,1448, ACE2(j),987,1302,1430,1431,1442,1445 , TMPRSS2(n),1430, nucleocapsid(k),1140, helicase(m),1140,1434,1439, endoribonuclease(p),1443, NSP16/10(q),1414, cathepsin L(r),1433, Wnt-3(s),1430, FZD(t),1430, LRP6(u),1430, ezrin(v),1444, ADRP(w),989, NRP1(x),1445, EP300(y),1424, PTGS2(z),1431, HSP90AA1(aa),1424,1431, matrix metalloproteinase 9(ab),1436, IL-6(ac),1429,1440, IL-10(ad),1429, VEGFA(ae),1440, and RELA(af),1440 proteins, and inhibition of spike-ACE2 interaction(o),1410.
In Vitro studies demonstrate inhibition of the Mpro(g),1325,1423,1450,1454 protein, and inhibition of spike-ACE2 interaction(o),1313.
Animal studies demonstrate efficacy in K18-hACE2 mice(ag),1457, db/db mice(ah),1455,1462, BALB/c mice(ai),1461, and rats1427.
Quercetin reduced proinflammatory cytokines and protected lung and kidney tissue against LPS-induced damage in mice1461, inhibits LPS-induced cytokine storm by modulating key inflammatory and antioxidant pathways in macrophages1413, and inhibits SARS-CoV-2 ORF3a ion channel activity, which contributes to viral pathogenicity and cytotoxicity1320.
12 quercetin clinical
studies973-984 show
efficacy, confirmed in multiple additional meta analyses1464,1465.
Potential mechanisms include direct antiviral activity, antioxidant and
anti-inflammatory activity, and immune support.
COVID-19 efficacy may depend heavily on advanced formulations for improved bioavailability.
Protocols typically combine multiple
treatments. No single treatment is guaranteed to be effective and safe for a specific individual.
Leading evidence-based protocols combine multiple treatments.
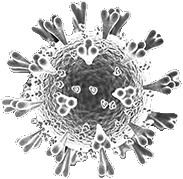
c19early.org
Combined treatments increase efficacy | |
---|---|
Monotherapy | 33% [30‑36%] |
Polytherapy | 68% [57‑77%] |
Complementary and
synergistic actions. There are many complementary mechanisms of action
across treatments, and studies show complementary and synergistic effects
with polytherapy81,1015,1178,1180,1186,1191,1466-1476 .
For example, Jitobaom et al.1180 showed >10x reduction in
IC50 with ivermectin and niclosamide, an RCT by Said et
al.1469 showed the combination of nigella sativa and vitamin D was
more effective than either alone, and an RCT by Wannigama et
al.919 showed improved results with fluvoxamine combined with
bromhexine, cyproheptadine, or niclosamide, compared to fluvoxamine alone.
Treatment efficacy may vary significantly across SARS-CoV-2 variants. For
example new variants may gain resistance to targeted
treatments1477-1483, and
the role of TMPRSS2 for cell entry differs across variants1484.
The efficacy of specific treatments varies depending on cell
type1485 due to differences in viral receptor expression, drug
distribution and metabolism, cell-specific mechanisms, and the relevance of
drug targets to specific cells.
Efficacy may also vary based on genetic
variants1486-1489.
Variable efficacy across SARS-CoV-2 variants, cell types, different tissues, and
host genetics, along with the complementary and synergistic actions of different
treatments, all point to greater efficacy with polytherapy.
In many studies, the standard of care given to all patients includes other
treatments—efficacy seen in these trials may rely in part on
synergistic effects.
Meta analysis of all early treatment trials shows 68% [57‑77%] lower risk
for studies using combined treatments, compared to 33% [30‑36%] for single
treatments.
SARS-CoV-2 evolution and the risk of
escape mutants suggests treatments with broader mechanisms of action and
polytherapy. SARS-CoV-2 can rapidly acquire mutations altering infectivity,
disease severity, and drug resistance even without selective
pressure1490. Antigenic drift can undermine more
variant-specific treatments like monoclonal antibodies and more specific
antivirals. Treatment with targeted antivirals may select for escape
mutations1491.
Less variant specific treatments and polytherapy targeting multiple viral and
host proteins may be more effective.
Systemic antivirals may be less
applicable to
low-risk infections. As SARS-CoV-2 has evolved, the frequency of
serious infections has reduced. Systemic antivirals may have a more limited
effect if infection does not spread beyond the upper respiratory tract.
Defined as ≥3 studies showing ≥10% improvement or >0% harm with statistical significance in meta analysis.
COPCOV has the largest number of treated patients of all HCQ/CQ RCTs.
The efficacy of endosomal entry inhibitors varies
across SARS-CoV-2 variants, depending on their reliance on the endosomal
pathway for entry. SARS-CoV-2 uses two major pathways for host cell entry:
endosomal entry via cathepsin proteases and TMPRSS2-mediated plasma membrane
fusion. Studies show increased reliance on TMPRSS2-mediated entry for the
Delta variant compared to the original strain. Conversely, Omicron variants
have shown significantly greater reliance on endosomal entry, suggesting
increased efficacy of endosomal acidification inhibitors. Specific
sub-variants may vary. A dual-inhibition strategy targeting both pathways
may be preferred.
When administered late in infection, HCQ may enhance viral egress by further increasing
lysosomal pH beyond the effect of ORF3a's water channel activity, thereby promoting
lysosomal exocytosis, inactivating degradative enzymes, and facilitating the release of
SARS-CoV-2 particles into the extracellular environment1033,1034.
The trimeric spike (S) protein is a glycoprotein that mediates viral entry by binding to the host ACE2 receptor, is critical for SARS-CoV-2's ability to infect host cells, and is a target of neutralizing antibodies. Inhibition of the spike protein prevents viral attachment, halting infection at the earliest stage.
The receptor binding domain is a specific region of the spike protein that binds ACE2 and is a major target of neutralizing antibodies. Focusing on the precise binding site allows highly specific disruption of viral attachment with reduced potential for off-target effects.
The main protease or Mpro, also known as 3CLpro or nsp5, is a cysteine protease that cleaves viral polyproteins into functional units needed for replication. Inhibiting Mpro disrupts the SARS-CoV-2 lifecycle within the host cell, preventing the creation of new copies.
RNA-dependent RNA polymerase (RdRp), also called nsp12, is the core enzyme of the viral replicase-transcriptase complex that copies the positive-sense viral RNA genome into negative-sense templates for progeny RNA synthesis. Inhibiting RdRp blocks viral genome replication and transcription.
The papain-like protease (PLpro) has multiple functions including cleaving viral polyproteins and suppressing the host immune response by deubiquitination and deISGylation of host proteins. Inhibiting PLpro may block viral replication and help restore normal immune responses.
The angiotensin converting enzyme 2 (ACE2) protein is a host cell transmembrane protein that serves as the cellular receptor for the SARS-CoV-2 spike protein. ACE2 is expressed on many cell types, including epithelial cells in the lungs, and allows the virus to enter and infect host cells. Inhibition may affect ACE2's physiological function in blood pressure control.
The nucleocapsid (N) protein binds and encapsulates the viral genome by coating the viral RNA. N enables formation and release of infectious virions and plays additional roles in viral replication and pathogenesis. N is also an immunodominant antigen used in diagnostic assays.
Non-structural protein 10 (nsp10) serves as an RNA chaperone and stabilizes conformations of nsp12 and nsp14 in the replicase-transcriptase complex, which synthesizes new viral RNAs. Nsp10 disruption may destabilize replicase-transcriptase complex activity.
The helicase, or nsp13, protein unwinds the double-stranded viral RNA, a crucial step in replication and transcription. Inhibition may prevent viral genome replication and the creation of new virus components.
Transmembrane protease serine 2 (TMPRSS2) is a host cell protease that primes the spike protein, facilitating cellular entry. TMPRSS2 activity helps enable cleavage of the spike protein required for membrane fusion and virus entry. Inhibition may especially protect respiratory epithelial cells, buy may have physiological effects.
The interaction between the SARS-CoV-2 spike protein and the human ACE2 receptor is a primary method of viral entry, inhibiting this interaction can prevent the virus from attaching to and entering host cells, halting infection at an early stage.
The endoribonuclease, also known as NendoU or nsp15, cleaves specific sequences in viral RNA which may help the virus evade detection by the host immune system. Inhibition may hinder the virus's ability to mask itself from the immune system, facilitating a stronger immune response.
The NSP16/10 complex consists of non-structural proteins 16 and 10, forming a 2'-O-methyltransferase that modifies the viral RNA cap structure. This modification helps the virus evade host immune detection by mimicking host mRNA, making NSP16/10 a promising antiviral target.
Cathepsin L is a host lysosomal cysteine protease that can prime the spike protein through an alternative pathway when TMPRSS2 is unavailable. Dual targeting of cathepsin L and TMPRSS2 may maximize disruption of alternative pathways for virus entry.
Wingless-related integration site (Wnt) ligand 3 is a host signaling molecule that activates the Wnt signaling pathway, which is important in development, cell growth, and tissue repair. Some studies suggest that SARS-CoV-2 infection may interfere with the Wnt signaling pathway, and that Wnt3a is involved in SARS-CoV-2 entry.
The frizzled (FZD) receptor is a host transmembrane receptor that binds Wnt ligands, initiating the Wnt signaling cascade. FZD serves as a co-receptor, along with ACE2, in some proposed mechanisms of SARS-CoV-2 infection. The virus may take advantage of this pathway as an alternative entry route.
Low-density lipoprotein receptor-related protein 6 is a cell surface co-receptor essential for Wnt signaling. LRP6 acts in tandem with FZD for signal transduction and has been discussed as a potential co-receptor for SARS-CoV-2 entry.
The ezrin protein links the cell membrane to the cytoskeleton (the cell's internal support structure) and plays a role in cell shape, movement, adhesion, and signaling. Drugs that occupy the same spot on ezrin where the viral spike protein would bind may hindering viral attachment, and drug binding could further stabilize ezrin, strengthening its potential natural capacity to impede viral fusion and entry.
The Adipocyte Differentiation-Related Protein (ADRP, also known as Perilipin 2 or PLIN2) is a lipid droplet protein regulating the storage and breakdown of fats in cells. SARS-CoV-2 may hijack the lipid handling machinery of host cells and ADRP may play a role in this process. Disrupting ADRP's interaction with the virus may hinder the virus's ability to use lipids for replication and assembly.
Neuropilin-1 (NRP1) is a cell surface receptor with roles in blood vessel development, nerve cell guidance, and immune responses. NRP1 may function as a co-receptor for SARS-CoV-2, facilitating viral entry into cells. Blocking NRP1 may disrupt an alternative route of viral entry.
EP300 (E1A Binding Protein P300) is a transcriptional coactivator involved in several cellular processes, including growth, differentiation, and apoptosis, through its acetyltransferase activity that modifies histones and non-histone proteins. EP300 facilitates viral entry into cells and upregulates inflammatory cytokine production.
Prostaglandin G/H synthase 2 (PTGS2, also known as COX-2) is an enzyme crucial for the production of inflammatory molecules called prostaglandins. PTGS2 plays a role in the inflammatory response that can become severe in COVID-19 and inhibitors (like some NSAIDs) may have benefits in dampening harmful inflammation, but note that prostaglandins have diverse physiological functions.
Heat Shock Protein 90 Alpha Family Class A Member 1 (HSP90AA1) is a chaperone protein that helps other proteins fold correctly and maintains their stability. HSP90AA1 plays roles in cell signaling, survival, and immune responses. HSP90AA1 may interact with numerous viral proteins, but note that it has diverse physiological functions.
Matrix metalloproteinase 9 (MMP9), also called gelatinase B, is a zinc-dependent enzyme that breaks down collagen and other components of the extracellular matrix. MMP9 levels increase in severe COVID-19. Overactive MMP9 can damage lung tissue and worsen inflammation. Inhibition of MMP9 may prevent excessive tissue damage and help regulate the inflammatory response.
The interleukin-6 (IL-6) pro-inflammatory cytokine (signaling molecule) has a complex role in the immune response and may trigger and perpetuate inflammation. Elevated IL-6 levels are associated with severe COVID-19 cases and cytokine storm. Anti-IL-6 therapies may be beneficial in reducing excessive inflammation in severe COVID-19 cases.
The interleukin-10 (IL-10) anti-inflammatory cytokine helps regulate and dampen immune responses, preventing excessive inflammation. IL-10 levels can also be elevated in severe COVID-19. IL-10 could either help control harmful inflammation or potentially contribute to immune suppression.
Vascular Endothelial Growth Factor A (VEGFA) promotes the growth of new blood vessels (angiogenesis) and has roles in inflammation and immune responses. VEGFA may contribute to blood vessel leakiness and excessive inflammation associated with severe COVID-19.
RELA is a transcription factor subunit of NF-kB and is a key regulator of inflammation, driving pro-inflammatory gene expression. SARS-CoV-2 may hijack and modulate NF-kB pathways.
A mouse model expressing the human ACE2 receptor under the control of the K18 promoter.
A mouse model of obesity and severe insulin resistance leading to type 2 diabetes due to a mutation in the leptin receptor gene that impairs satiety signaling.
A mouse model commonly used in infectious disease and cancer research due to higher immune response and susceptibility to infection.
Dugied et al., Multimodal SARS-CoV-2 interactome sketches the virus-host spatial organization, Communications Biology, doi:10.1038/s42003-025-07933-z.
Malone et al., Structures and functions of coronavirus replication–transcription complexes and their relevance for SARS-CoV-2 drug design, Nature Reviews Molecular Cell Biology, doi:10.1038/s41580-021-00432-z.
Murigneux et al., Proteomic analysis of SARS-CoV-2 particles unveils a key role of G3BP proteins in viral assembly, Nature Communications, doi:10.1038/s41467-024-44958-0.
Lv et al., Host proviral and antiviral factors for SARS-CoV-2, Virus Genes, doi:10.1007/s11262-021-01869-2.
Lui et al., Nsp1 facilitates SARS-CoV-2 replication through calcineurin-NFAT signaling, Virology, doi:10.1128/mbio.00392-24.
Niarakis et al., Drug-target identification in COVID-19 disease mechanisms using computational systems biology approaches, Frontiers in Immunology, doi:10.3389/fimmu.2023.1282859.
Katiyar et al., SARS-CoV-2 Assembly: Gaining Infectivity and Beyond, Viruses, doi:10.3390/v16111648.
Wu et al., Decoding the genome of SARS-CoV-2: a pathway to drug development through translation inhibition, RNA Biology, doi:10.1080/15476286.2024.2433830.
Chowdhury et al., A Comparative Study on Ivermectin-Doxycycline and Hydroxychloroquine-Azithromycin Therapy on COVID-19 Patients, Eurasian Journal of Medicine and Oncology, doi:10.14744/ejmo.2021.16263.
Espitia-Hernandez et al., Effects of Ivermectin-azithromycin-cholecalciferol combined therapy on COVID-19 infected patients: A proof of concept study, Biomedical Research, 31:5, www.biomedres.info/biomedical-research/effects-of-ivermectinazithromycincholecalciferol-combined-therapy-on-covid19-infected-patients-a-proof-of-concept-study-14435.html.
Carvallo et al., Safety and Efficacy of the Combined Use of Ivermectin, Dexamethasone, Enoxaparin and Aspirina against COVID-19 the I.D.E.A. Protocol, Journal of Clinical Trials, 11:459 (preprint 9/15/20), www.longdom.org/open-access/safety-and-efficacy-of-the-combined-use-of-ivermectin-dexamethasone-enoxaparin-and-aspirina-against-covid19-the-idea-protocol-70290.html.
Mahmud et al., Ivermectin in combination with doxycycline for treating COVID-19 symptoms: a randomized trial, Journal of International Medical Research, doi:10.5061/dryad.qjq2bvqf6.
Szente Fonseca et al., Risk of Hospitalization for Covid-19 Outpatients Treated with Various Drug Regimens in Brazil: Comparative Analysis, Travel Medicine and Infectious Disease, doi:10.1016/j.tmaid.2020.101906.
Cadegiani et al., Early COVID-19 Therapy with azithromycin plus nitazoxanide, ivermectin or hydroxychloroquine in Outpatient Settings Significantly Improved COVID-19 outcomes compared to Known outcomes in untreated patients, New Microbes and New Infections, doi:10.1016/j.nmni.2021.100915.
Ahmed et al., A five day course of ivermectin for the treatment of COVID-19 may reduce the duration of illness, International Journal of Infectious Diseases, doi:10.1016/j.ijid.2020.11.191.
Chaccour et al., The effect of early treatment with ivermectin on viral load, symptoms and humoral response in patients with non-severe COVID-19: A pilot, double-blind, placebo-controlled, randomized clinical trial, eClinicalMedicine, doi:10.1016/j.eclinm.2020.100720.
Ghauri et al., Ivermectin Use Associated with Reduced Duration of Covid-19 Febrile Illness in a Community Setting, International Journal of Clinical Studies & Medical Case Reports, doi:10.46998/IJCMCR.2021.13.000320.
Babalola et al., Ivermectin shows clinical benefits in mild to moderate COVID19: A randomised controlled double-blind, dose-response study in Lagos, QJM: An International Journal of Medicine, doi:10.1093/qjmed/hcab035.
Ravikirti et al., Ivermectin as a potential treatment for mild to moderate COVID-19: A double blind randomized placebo-controlled trial, Journal of Pharmacy & Pharmaceutical Sciences, doi:10.18433/jpps32105.
Bukhari et al., Efficacy of Ivermectin in COVID-19 Patients with Mild to Moderate Disease, medRxiv, doi:10.1101/2021.02.02.21250840.
Mohan et al., Single-dose oral ivermectin in mild and moderate COVID-19 (RIVET-COV): a single-centre randomized, placebo-controlled trial, Journal of Infection and Chemotherapy, doi:10.1016/j.jiac.2021.08.021.
Biber et al., The effect of ivermectin on the viral load and culture viability in early treatment of non-hospitalized patients with mild COVID-19 – A double-blind, randomized placebo-controlled trial, International Journal of Infectious Diseases, doi:10.1016/j.ijid.2022.07.003.
Elalfy et al., Effect of a combination of Nitazoxanide, Ribavirin and Ivermectin plus zinc supplement (MANS.NRIZ study) on the clearance of mild COVID-1, Journal of Medical Virology, doi:10.1002/jmv.26880.
López-Medina et al., Effect of Ivermectin on Time to Resolution of Symptoms Among Adults With Mild COVID-19: A Randomized Clinical Trial, JAMA, doi:10.1001/jama.2021.3071.
Roy et al., Outcome of Different Therapeutic Interventions in Mild COVID-19 Patients in a Single OPD Clinic of West Bengal: A Retrospective study, medRxiv, doi:10.1101/2021.03.08.21252883.
Chahla et al., Randomized trials - Ivermectin repurposing for COVID-19 treatment of outpatients with mild disease in primary health care centers, Research, Society and Development, doi:10.33448/rsd-v11i8.30844.
Mourya et al., Comparative Analytical Study of Two Different Drug Regimens in Treatment of Covid 19 Positive Patients in Index Medical College Hospital and Research Center, Indore, India, International Journal of Health and Clinical Research, 4:6, ijhcr.com/index.php/ijhcr/article/view/1263.
Loue et al., Ivermectin and COVID-19 in Care Home: Case Report, J. Infectious Diseases and Epidemiology, doi:10.23937/2474-3658/1510202.
Merino et al., Ivermectin and the odds of hospitalization due to COVID-19: evidence from a quasi-experimental analysis based on a public intervention in Mexico City, Preprint, web.archive.org/web/20211218011849/https://files.osf.io/v1/resources/r93g4/providers/osfstorage/609085945533b4031ee1c789?action=download&direct&version=1.
Faisal et al., Potential use of azithromycin alone and in combination with ivermectin in fighting against the symptoms of COVID-19, The Professional Medical Journal, doi:10.29309/TPMJ/2021.28.05.5867.
Aref et al., Clinical, Biochemical and Molecular Evaluations of Ivermectin Mucoadhesive Nanosuspension Nasal Spray in Reducing Upper Respiratory Symptoms of Mild COVID-19, International Journal of Nanomedicine, doi:10.2147/IJN.S313093.
Krolewiecki et al., Antiviral effect of high-dose ivermectin in adults with COVID-19: A proof-of-concept randomized trial, eClinicalMedicine, doi:10.1016/j.eclinm.2021.100959.
Vallejos et al., Ivermectin to prevent hospitalizations in patients with COVID-19 (IVERCOR-COVID19) a randomized, double-blind, placebo-controlled trial, BMC Infectious Diseases, doi:10.1186/s12879-021-06348-5.
Reis et al., Effect of Early Treatment with Ivermectin among Patients with Covid-19, New England Journal of Medicine, doi:10.1056/NEJMoa2115869.
Buonfrate et al., High dose ivermectin for the early treatment of COVID-19 (COVER study): a randomised, double-blind, multicentre, phase II, dose-finding, proof of concept trial, International Journal of Antimicrobial Agents, doi:10.1016/j.ijantimicag.2021.106516.
Mayer et al., Safety and Efficacy of a MEURI Program for the Use of High Dose Ivermectin in COVID-19 Patients, Frontiers in Public Health, doi:10.3389/fpubh.2022.813378.
Borody et al., Combination Therapy For COVID-19 Based on Ivermectin in an Australian Population, TrialSite News, www.trialsitenews.com/a/combination-therapy-for-covid-19-based-on-ivermectin-in-an-australian-population.
Abbas et al., The Effect of Ivermectin on Reducing Viral Symptoms in Patients with Mild COVID-19, Indian Journal of Pharmaceutical Sciences, doi:10.36468/pharmaceutical-sciences.spl.416.
de Jesús Ascencio-Montiel et al., A Multimodal Strategy to Reduce the Risk of Hospitalization/death in Ambulatory Patients with COVID-19, Archives of Medical Research, doi:10.1016/j.arcmed.2022.01.002.
Manomaipiboon et al., Efficacy and safety of ivermectin in the treatment of mild-to-moderate COVID-19 infection: A randomized, double blind, placebo, controlled trial, Trials, doi:10.1186/s13063-022-06649-3.
de la Rocha et al., Ivermectin compared with placebo in the clinical course in Mexican patients with asymptomatic and mild COVID-19: a randomized clinical trial, BMC Infectious Diseases, doi:10.1186/s12879-022-07890-6.
Rezai et al., Non-effectiveness of Ivermectin on Inpatients and Outpatients With COVID-19; Results of Two Randomized, Double-Blinded, Placebo-Controlled Clinical Trials, Frontiers in Medicine, doi:10.3389/fmed.2022.919708.
Mirahmadizadeh et al., Efficacy of single-dose and double-dose ivermectin early treatment in preventing progression to hospitalization in mild COVID-19: A multi-arm, parallel-group randomized, double-blind, placebo-controlled trial, Respirology, doi:10.1111/resp.14318.
Schilling et al., Pharmacometrics of high dose ivermectin in early COVID-19: an open label, randomized, controlled adaptive platform trial (PLATCOV), eLife, doi:10.7554/eLife.83201.
Bramante et al., Randomized Trial of Metformin, Ivermectin, and Fluvoxamine for Covid-19, NEJM, doi:10.1056/NEJMoa2201662.
Mikamo et al., Efficacy and safety of ivermectin in patients with mild COVID-19 in Japan and Thailand, Journal of Infection and Chemotherapy, doi:10.1016/j.jiac.2023.12.012.
Siripongboonsitti et al., A Randomized Trial to Assess the Acceleration of Viral Clearance by the Combination Favipiravir/Ivermectin/Niclosamide in Mild-to-Moderate COVID-19 Adult Patients (FINCOV), Journal of Infection and Public Health, doi:10.1016/j.jiph.2024.03.030.
Wijewickrema et al., Efficacy and safety of oral ivermectin in the treatment of mild to moderate Covid-19 patients: a multi-centre double-blind randomized controlled clinical trial, BMC Infectious Diseases, doi:10.1186/s12879-024-09563-y.
Gorial et al., Effectiveness of Ivermectin as add-on Therapy in COVID-19 Management (Pilot Trial), medRxiv, doi:10.1101/2020.07.07.20145979.
Kishoria et al., Ivermectin as adjuvant to hydroxychloroquine in patients resistant to standard treatment for SARS-CoV-2: results of an open-label randomized clinical study, Paripex - Indian Journal of Research, doi:10.36106/paripex/4801859.
Podder et al., Outcome of ivermectin treated mild to moderate COVID-19 cases: a single-centre, open-label, randomised controlled study, IMC J. Med. Science, doi:10.3329/imcjms.v14i2.52826.
Khan et al., Ivermectin treatment may improve the prognosis of patients with COVID-19, Archivos de Bronconeumología, doi:10.1016/j.arbres.2020.08.007.
Chachar et al., Effectiveness of Ivermectin in SARS-CoV-2/COVID-19 Patients, International Journal of Sciences, 9:31-35, doi:10.18483/ijSci.2378.
Soto-Becerra et al., Real-World Effectiveness of hydroxychloroquine, azithromycin, and ivermectin among hospitalized COVID-19 patients: Results of a target trial emulation using observational data from a nationwide Healthcare System in Peru, medRxiv, doi:10.1101/2020.10.06.20208066.
Rajter et al., Use of Ivermectin is Associated with Lower Mortality in Hospitalized Patients with COVID-19 (ICON study), Chest, doi:10.1016/j.chest.2020.10.009.
Hashim et al., Controlled randomized clinical trial on using Ivermectin with doxycycline for treating COVID-19 patients in Baghdad, Iraq, Iraqi Journal of Medical Science, 19:1, www.iraqijms.net/upload/pdf/iraqijms60db8b76d3b1e.pdf.
Camprubí et al., Lack of efficacy of standard doses of ivermectin in severe COVID-19 patients, PLoS ONE, 15:11, doi:10.1371/journal.pone.0242184.
Spoorthi et al., Utility of Ivermectin and Doxycycline combination for the treatment of SARSCoV-2, IAIM, 2020, 7:10, 177-182, iaimjournal.com/wp-content/uploads/2020/10/iaim_2020_0710_23.pdf.
Budhiraja et al., Clinical Profile of First 1000 COVID-19 Cases Admitted at Tertiary Care Hospitals and the Correlates of their Mortality: An Indian Experience, medRxiv, doi:10.1101/2020.11.16.20232223.
Okumuş et al., Evaluation of the Effectiveness and Safety of Adding Ivermectin to Treatment in Severe COVID-19 Patients, BMC Infectious Diseases, doi:10.1186/s12879-021-06104-9.
Shahbaznejad et al., Effects of Ivermectin in Patients With COVID-19: A Multicenter, Double-blind, Randomized, Controlled Clinical Trial, Clinical Therapeutics, doi:10.1016/j.clinthera.2021.04.007.
Lima-Morales et al., Effectiveness of a multidrug therapy consisting of ivermectin, azithromycin, montelukast and acetylsalicylic acid to prevent hospitalization and death among ambulatory COVID-19 cases in Tlaxcala, Mexico, International Journal of Infectious Diseases, doi:10.1016/j.ijid.2021.02.014.
Beltran Gonzalez et al., Efficacy and Safety of Ivermectin and Hydroxychloroquine in Patients with Severe COVID-19: A Randomized Controlled Trial, Infectious Disease Reports, doi:10.3390/idr14020020.
Pott-Junior et al., Use of ivermectin in the treatment of Covid-19: a pilot trial, Toxicology Reports, doi:10.1016/j.toxrep.2021.03.003.
Huvemek, Kovid-19 - Huvemek® Phase 2 clinical trial, Press Release, huvemec.bg/covid-19-huvemec-klinichno-izpitanie/za-isledvaneto/.
Ahsan et al., Clinical Variants, Characteristics, and Outcomes Among COVID-19 Patients: A Case Series Analysis at a Tertiary Care Hospital in Karachi, Pakistan, Cureus, doi:10.7759/cureus.14761.
Abd-Elsalam et al., Clinical Study Evaluating the Efficacy of Ivermectin in COVID-19 Treatment: A Randomized Controlled Study, Journal of Medical Virology, doi:10.1002/jmv.27122.
Hazan et al., Effectiveness of ivermectin-based multidrug therapy in severely hypoxic, ambulatory COVID-19 patients, medRxiv, doi:10.1101/2021.07.06.21259924.
Elavarasi et al., Clinical features, demography, and predictors of outcomes of SARS-CoV-2 infection at a tertiary care hospital in India: A cohort study, Lung India, doi:10.4103/lungindia.lungindia_493_21.
Rezk et al., miRNA-223-3p, miRNA- 2909 and Cytokines Expression in COVID-19 Patients Treated with Ivermectin, Zagazig University Medical Journal, doi:10.21608/zumj.2021.92746.2329.
Lim et al., Efficacy of Ivermectin Treatment on Disease Progression Among Adults With Mild to Moderate COVID-19 and Comorbidities: The I-TECH Randomized Clinical Trial, JAMA, doi:10.1001/jamainternmed.2022.0189.
Ozer et al., Effectiveness and Safety of Ivermectin in COVID-19 Patients: A Prospective Study at A Safety-Net Hospital, Journal of Medical Virology, doi:10.1002/jmv.27469.
Ferreira et al., Outcomes associated with Hydroxychloroquine and Ivermectin in hospitalized patients with COVID-19: a single-center experience, Revista da Associação Médica Brasileira, doi:10.1590/1806-9282.20210661.
Jamir et al., Determinants of Outcome Among Critically Ill Police Personnel With COVID-19: A Retrospective Observational Study From Andhra Pradesh, India, Cureus, doi:10.7759/cureus.20394.
Baguma et al., Characteristics of the COVID-19 patients treated at Gulu Regional Referral Hospital, Northern Uganda: A cross-sectional study, Research Square, doi:10.21203/rs.3.rs-1193578/v1.
Mustafa et al., Pattern of medication utilization in hospitalized patients with COVID-19 in three District Headquarters Hospitals in the Punjab province of Pakistan, Exploratory Research in Clinical and Social Pharmacy, doi:10.1016/j.rcsop.2021.100101.
Shimizu et al., Ivermectin administration is associated with lower gastrointestinal complications and greater ventilator-free days in ventilated patients with COVID-19: A propensity score analysis, Journal of Infection and Chemotherapy, doi:10.1016/j.jiac.2021.12.024.
Zubair et al., The effect of ivermectin on non-severe and severe COVID-19 disease and gender-based difference of its effectiveness, Monaldi Archives for Chest Disease, doi:10.4081/monaldi.2022.2062.
Thairu et al., A Comparison of Ivermectin and Non Ivermectin Based Regimen for COVID-19 in Abuja: Effects on Virus Clearance, Days-to-discharge and Mortality, Journal of Pharmaceutical Research International, doi:10.9734/jpri/2022/v34i44A36328.
Efimenko et al., Treatment with Ivermectin Is Associated with Decreased Mortality in COVID-19 Patients: Analysis of a National Federated Database, International Journal of Infectious Diseases, doi:10.1016/j.ijid.2021.12.096.
Soto et al., Mortality and associated risk factors in patients hospitalized due to COVID-19 in a Peruvian reference hospital, PLOS ONE, doi:10.1371/journal.pone.0264789.
Ravikirti (B) et al., Association between Ivermectin treatment and mortality in Covid-19: A hospital-based case-control study, Research Square, doi:10.21203/rs.3.rs-1522422/v1.
George et al., Single Dose of Ivermectin is not Useful in Patients with Hematological Disorders and COVID-19 Illness: A Phase II B Open Labelled Randomized Controlled Trial, Indian Journal of Hematology and Blood Transfusion, doi:10.1007/s12288-022-01546-w.
Naggie et al., Effect of Ivermectin vs Placebo on Time to Sustained Recovery in Outpatients With Mild to Moderate COVID-19: A Randomized Clinical Trial, JAMA, doi:10.1001/jama.2022.18590.
Rezai (B) et al., Non-effectiveness of Ivermectin on Inpatients and Outpatients With COVID-19; Results of Two Randomized, Double-Blinded, Placebo-Controlled Clinical Trials, Frontiers in Medicine, doi:10.3389/fmed.2022.919708.
Qadeer et al., Ivermectin A Potential Treatment In Covid-19, Related to Critical Illness, Pakistan Journal of Medical and Health Sciences, doi:10.53350/pjmhs2216824.
Aref (B) et al., Possible Role of Ivermectin Mucoadhesive Nanosuspension Nasal Spray in Recovery of Post-COVID-19 Anosmia, Infection and Drug Resistance, doi:10.2147/IDR.S381715.
Ochoa-Jaramillo et al., Clinical efficacy and safety of ivermectin (400 μg/kg, single dose) in patients with severe COVID-19: a randomized clinical trial, Revista Infectio, doi:10.22354/24223794.1105.
Sarojvisut et al., An Open Label Randomized Controlled Trial of Ivermectin Plus Favipiravir-Based Standard of Care versus Favipiravir-Based Standard of Care for Treatment of Moderate COVID-19 in Thailand, Infection & Chemotherapy, doi:10.3947/ic.2022.0127.
Munir et al., Clinical Disease Characteristics and Treatment Trajectories Associated with Mortality among COVID-19 Patients in Punjab, Pakistan, Healthcare, doi:10.3390/healthcare11081192.
Llenas-García et al., Ivermectin Effect on In-Hospital Mortality and Need for Respiratory Support in COVID-19 Pneumonia: Propensity Score-Matched Retrospective Study, Viruses, doi:10.3390/v15051138.
Wada et al., Efficacy and safety of single-dose ivermectin in mild-to-moderate COVID-19: the double-blind, randomized, placebo-controlled CORVETTE-01 trial, Frontiers in Medicine, doi:10.3389/fmed.2023.1139046.
Osati et al., Clinical manifestations and mortality among hospitalized COVID-19 patients in Tanzania, 2021-2022., medRxiv, doi:10.1101/2023.07.13.23292643.
Hayward et al., Ivermectin for COVID-19 in adults in the community (PRINCIPLE): an open, randomised, controlled, adaptive platform trial of short- and longer-term outcomes, Journal of Infection, doi:10.1016/j.jinf.2024.106130.
Varnaseri et al., Ivermectin as a Potential Addition to the Limited Anti-COVID-19 Arsenal: A Double-Blinded Clinical Trial, Jundishapur Journal of Health Sciences, doi:10.5812/jjhs-146703.
Hashmi et al., Ivermectin for patients admitted to an ICU with COVID-19: REMAP-CAP randomized controlled trial, Critical Care Reviews, CCR24, criticalcarereviews.com/meetings/ccr24#1.
Shouman et al., Use of Ivermectin as a Potential Chemoprophylaxis for COVID-19 in Egypt: A Randomised Clinical Trial, Journal of Clinical and Diagnostic Research, doi:10.7860/JCDR/2021/46795.14529.
Carvallo (B) et al., Usefulness of Topic Ivermectin and Carrageenan to Prevent Contagion of Covid 19 (IVERCAR), NCT04425850, clinicaltrials.gov/ct2/show/results/NCT04425850.
Behera et al., Role of ivermectin in the prevention of SARS-CoV-2 infection among healthcare workers in India: A matched case-control study, PLOS ONE, doi:10.1371/journal.pone.0247163.
Carvallo (C) et al., Study of the Efficacy and Safety of Topical Ivermectin + Iota-Carrageenan in the Prophylaxis against COVID-19 in Health Personnel, Journal of Biomedical Research and Clinical Investigation, doi:10.31546/2633-8653.1007.
Hellwig et al., A COVID-19 Prophylaxis? Lower incidence associated with prophylactic administration of Ivermectin, International Journal of Antimicrobial Agents, doi:10.1016/j.ijantimicag.2020.106248.
Bernigaud et al., Ivermectin benefit: from scabies to COVID-19, an example of serendipity, Annals of Dermatology and Venereology, doi:10.1016/j.annder.2020.09.231.
Alam et al., Ivermectin as Pre-exposure Prophylaxis for COVID-19 among Healthcare Providers in a Selected Tertiary Hospital in Dhaka – An Observational Study, European Journal of Medical and Health Sciences, doi:10.24018/ejmed.2020.2.6.599.
IVERCOR PREP, Ivermectina en agentes de salud e IVERCOR COVID19, Preliminary Results, web.archive.org/web/20210226215453/https://twitter.com/Covid19Crusher/status/1365420061859717124.
Chahla (B) et al., Intensive Treatment With Ivermectin and Iota-Carrageenan as Pre-exposure Prophylaxis for COVID-19 in Health Care Workers From Tucuman, Argentina, American Journal of Therapeutics, doi:10.1097/MJT.0000000000001433.
Behera (B) et al., Prophylactic Role of Ivermectin in Severe Acute Respiratory Syndrome Coronavirus 2 Infection Among Healthcare Workers, Cureus, doi:10.7759/cureus.16897.
Tanioka et al., Why COVID-19 is not so spread in Africa: How does Ivermectin affect it?, medRxiv, doi:10.1101/2021.03.26.21254377.
Seet et al., Positive impact of oral hydroxychloroquine and povidone-iodine throat spray for COVID-19 prophylaxis: an open-label randomized trial, International Journal of Infectious Diseases, doi:10.1016/j.ijid.2021.04.035.
Morgenstern et al., Ivermectin as a SARS-CoV-2 Pre-Exposure Prophylaxis Method in Healthcare Workers: A Propensity Score-Matched Retrospective Cohort Study, Cureus, doi:10.7759/cureus.17455.
Mondal et al., Prevalence of COVID-19 Infection and Identification of Risk Factors among Asymptomatic Healthcare Workers: A Serosurvey Involving Multiple Hospitals in West Bengal, Journal of the Indian Medical Association, 119:5, onlinejima.com/read_journals.php?article=683.
Samajdar et al., Ivermectin and Hydroxychloroquine for Chemo-Prophylaxis of COVID-19: A Questionnaire Survey of Perception and Prescribing Practice of Physicians vis-a-vis Outcomes, Journal of the Association of Physicians India, 69:11, www.researchgate.net/publication/356294136_Ivermectin_and_Hydroxychloroquine_for_Chemo-Prophylaxis_of_COVID-19_A_Questionnaire_Survey_of_Perception_and_Prescribing_Practice_of_Physicians_vis-a-vis_Outcomes.
Kerr et al., Ivermectin Prophylaxis Used for COVID-19: A Citywide, Prospective, Observational Study of 223,128 Subjects Using Propensity Score Matching, Cureus, doi:10.7759/cureus.21272.
Desort-Henin et al., The SAIVE Trial, Post-Exposure use of ivermectin in Covid-19 prevention: Efficacy and Safety Results, ECCMID 2023 (results released 1/5/2023), www.medincell.com/wp-content/uploads/2024/03/Poster-SAIVE-April2023-OK3.pdf.
Esper et al., Empirical treatment with hydroxychloroquine and azithromycin for suspected cases of COVID-19 followed-up by telemedicine, Prevent Senior Institute, São Paulo, Brazil, pgibertie.com/wp-content/uploads/2020/04/2020.04.15-journal-manuscript-final.pdf.
Ashraf et al., COVID-19 in Iran, a comprehensive investigation from exposure to treatment outcomes, medRxiv doi:10.1101/2020.04.20.20072421, www.researchgate.net/publication/341197843_COVID-19_in_Iran_a_comprehensive_investigation_from_exposure_to_treatment_outcomes.
Huang et al., Preliminary evidence from a multicenter prospective observational study of the safety and efficacy of chloroquine for the treatment of COVID-19, National Science Review, doi:10.1093/nsr/nwaa113.
Guérin et al., Azithromycin and Hydroxychloroquine Accelerate Recovery of Outpatients with Mild/Moderate COVID-19, Asian Journal of Medicine and Health, doi:10.9734/ajmah/2020/v18i730224.
Derwand et al., COVID-19 Outpatients – Early Risk-Stratified Treatment with Zinc Plus Low Dose Hydroxychloroquine and Azithromycin: A Retrospective Case Series Study, International Journal of Antimicrobial Agents, doi:10.1016/j.ijantimicag.2020.106214.
Smith et al., Evaluating the Efficacy of Hydroxychloroquine and Azithromycin to Prevent Hospitalization or Death in Persons With COVID-19, NCT04358068, clinicaltrials.gov/study/NCT04358068.
Mitjà et al., Hydroxychloroquine for Early Treatment of Adults with Mild Covid-19: A Randomized-Controlled Trial, Clinical Infectious Diseases, ciaa1009, doi:10.1093/cid/ciaa1009.
Skipper et al., Hydroxychloroquine in Nonhospitalized Adults With Early COVID-19: A Randomized Trial, Annals of Internal Medicine, doi:10.7326/M20-4207.
Hong et al., Early Hydroxychloroquine Administration for Rapid Severe Acute Respiratory Syndrome Coronavirus 2 Eradication, Infection & Chemotherapy, 2020, doi:10.3947/ic.2020.52.3.396.
Bernabeu-Wittel et al., Effectiveness of a On-Site Medicalization Program for Nursing Homes with COVID-19 Outbreaks, J. Gerontol. A Biol. Sci. Med. Sci., doi:10.1093/gerona/glaa192.
Yu et al., Beneficial effects exerted by hydroxychloroquine in treating COVID-19 patients via protecting multiple organs, Science China Life Sciences, 2020 Aug 3, doi:10.1007/s11427-020-1782-1.
Ly et al., Pattern of SARS-CoV-2 infection among dependant elderly residents living in retirement homes in Marseille, France, March-June 2020, International Journal of Antimicrobial Agents, doi:10.1016/j.ijantimicag.2020.106219.
Ip et al., Hydroxychloroquine in the treatment of outpatients with mildly symptomatic COVID-19: A multi-center observational study, BMC Infectious Diseases, doi:10.1186/s12879-021-05773-w.
Heras et al., COVID-19 mortality risk factors in older people in a long-term care center, European Geriatric Medicine, doi:10.1007/s41999-020-00432-w.
Kirenga et al., Characteristics and outcomes of admitted patients infected with SARS-CoV-2 in Uganda, BMJ Open Respiratory Research, doi:10.1136/bmjresp-2020-000646.
Sulaiman et al., The Effect of Early Hydroxychloroquine-based Therapy in COVID-19 Patients in Ambulatory Care Settings: A Nationwide Prospective Cohort Study, medRxiv, doi:10.1101/2020.09.09.20184143.
Guisado-Vasco et al., Clinical characteristics and outcomes among hospitalized adults with severe COVID-19 admitted to a tertiary medical center and receiving antiviral, antimalarials, glucocorticoids, or immunomodulation with tocilizumab or cyclosporine: A retrospective observational study (COQUIMA cohort), eClinicalMedicine, doi:10.1016/j.eclinm.2020.100591.
Szente Fonseca (B) et al., Risk of Hospitalization for Covid-19 Outpatients Treated with Various Drug Regimens in Brazil: Comparative Analysis, Travel Medicine and Infectious Disease, doi:10.1016/j.tmaid.2020.101906.
Cadegiani (B) et al., Early COVID-19 Therapy with azithromycin plus nitazoxanide, ivermectin or hydroxychloroquine in Outpatient Settings Significantly Improved COVID-19 outcomes compared to Known outcomes in untreated patients, New Microbes and New Infections, doi:10.1016/j.nmni.2021.100915.
Simova et al., Hydroxychloroquine for prophylaxis and treatment of COVID-19 in health care workers, New Microbes and New Infections, doi:10.1016/j.nmni.2020.100813.
Omrani et al., Randomized double-blinded placebo-controlled trial of hydroxychloroquine with or without azithromycin for virologic cure of non-severe Covid-19, eClinicalMedicine, doi:10.1016/j.eclinm.2020.100645.
Agusti et al., Efficacy and safety of hydroxychloroquine in healthcare professionals with mild SARS-CoV-2 infection: prospective, non-randomized trial, Enfermedades Infecciosas y Microbiología Clínica, doi:10.1016/j.eimc.2020.10.023.
Su et al., Efficacy of early hydroxychloroquine treatment in preventing COVID-19 pneumonia aggravation, the experience from Shanghai, China, BioScience Trends, doi:10.5582/bst.2020.03340.
Amaravadi et al., Hydroxychloroquine for SARS-CoV-2 positive patients quarantined at home: The first interim analysis of a remotely conducted randomized clinical trial, medRxiv, doi:10.1101/2021.02.22.21252228.
Roy (B) et al., Outcome of Different Therapeutic Interventions in Mild COVID-19 Patients in a Single OPD Clinic of West Bengal: A Retrospective study, medRxiv, doi:10.1101/2021.03.08.21252883.
Mokhtari et al., Clinical outcomes of patients with mild COVID-19 following treatment with hydroxychloroquine in an outpatient setting, International Immunopharmacology, doi:10.1016/j.intimp.2021.107636.
Corradini et al., Clinical factors associated with death in 3044 COVID-19 patients managed in internal medicine wards in Italy: results from the SIMI-COVID-19 study of the Italian Society of Internal Medicine (SIMI), Internal and Emergency Medicine, doi:10.1007/s11739-021-02742-8.
Million et al., Early Treatment with Hydroxychloroquine and Azithromycin in 10,429 COVID-19 Outpatients: A Monocentric Retrospective Cohort Study, Reviews in Cardiovascular Medicine, doi:10.31083/j.rcm2203116.
Sobngwi et al., Doxycycline vs Hydroxychloroquine + Azithromycin in the Management of COVID-19 Patients: An Open-Label Randomized Clinical Trial in Sub-Saharan Africa (DOXYCOV), Cureus, doi:10.7759/cureus.45619.
Rodrigues et al., Hydroxychloroquine plus azithromycin early treatment of mild COVID-19 in outpatient setting: a randomized, double-blinded, placebo-controlled clinical trial evaluating viral clearance, International Journal of Antimicrobial Agents, doi:10.1016/j.ijantimicag.2021.106428.
Sawanpanyalert et al., Assessment of outcomes following implementation of antiviral treatment guidelines for COVID-19 during the first wave in Thailand, Southeast Asian Journal of Tropical Medicine and Public Health, 52:4, journal.seameotropmednetwork.org/index.php/jtropmed/article/view/490.
Atipornwanich et al., Various Combinations of Favipiravir, Lopinavir-Ritonavir, Darunavir-Ritonavir, High-Dose Oseltamivir, and Hydroxychloroquine for the Treatment of COVID-19: A Randomized Controlled Trial (FIGHT-COVID-19 Study), SSRN Electronic Journal, doi:10.2139/ssrn.3936499.
Chechter et al., Evaluation of patients treated by telemedicine in the beginning of the COVID-19 pandemic in São Paulo, Brazil: A non-randomized clinical trial preliminary study, Heliyon, doi:10.1016/j.heliyon.2023.e15337.
Rouamba et al., Assessment of Recovery Time, Worsening and Death, among COVID-19 inpatients and outpatients, under treatment with Hydroxychloroquine or Chloroquine plus Azithromycin Combination in Burkina Faso, International Journal of Infectious Diseases, doi:10.1016/j.ijid.2022.02.034.
Avezum et al., Hydroxychloroquine versus placebo in the treatment of non-hospitalised patients with COVID-19 (COPE – Coalition V): A double-blind, multicentre, randomised, controlled trial, The Lancet Regional Health - Americas, doi:10.1016/j.lana.2022.100243.
Roy-García et al., Efficacy and Safety of Fixed Combination of Hydroxychloroquine with Azithromycin Versus Hydroxychloroquine and Placebo in Patients with Mild COVID-19: Randomized, double blind, Placebo controlled trial, medRxiv, doi:10.1101/2022.04.06.22273531.
Rathod et al., Risk Factors associated with COVID-19 Patients in India: A Single Center Retrospective Cohort Study, The Journal of the Association of Physicians of India, doi:10.5005/japi-11001-0263.
Azhar et al., Effectiveness of early pharmaceutical interventions in symptomatic COVID-19 patients: A randomized clinical trial, Pakistan Journal of Medical Sciences, doi:10.12669/pjms.40.5.8757.
Xia et al., Efficacy of Chloroquine and Lopinavir/ Ritonavir in mild/general novel coronavirus (CoVID-19) infections: a prospective, open-label, multicenter randomized controlled clinical study, ChiCTR2000029741, www.chictr.org.cn/showproj.aspx?proj=49263.
Chen et al., A pilot study of hydroxychloroquine in treatment of patients with common coronavirus disease-19 (COVID-19), J. Zhejiang University (Med Sci), doi:10.3785/j.issn.1008-9292.2020.03.03.
Zhong Nanshan (钟南山), Efficacy and safety of chloroquine for treatment of COVID-19. An open-label, multi-center, non-randomized trial, twitter.com/JamesTodaroMD/status/1243260720944480265.
Chen (B) et al., Efficacy of hydroxychloroquine in patients with COVID-19: results of a randomized clinical trial, medRxiv, doi:10.1101/2020.03.22.20040758.
Barbosa et al., Clinical outcomes of hydroxychloroquine in hospitalized patients with COVID-19: a quasi-randomized comparative study, Preprint, www.sefq.es/_pdfs/NEJM_Hydroxychlorquine.pdf.
Tang et al., Hydroxychloroquine in patients with mainly mild to moderate coronavirus disease 2019: open label, randomised controlled trial, BMJ 2020, 369, doi:10.1136/bmj.m1849.
Magagnoli et al., Outcomes of hydroxychloroquine usage in United States veterans hospitalized with Covid-19, Med (2020), doi:10.1016/j.medj.2020.06.001.
Auld et al., ICU and ventilator mortality among critically ill adults with COVID-19, Critical Care Medicine, doi:10.1097/ccm.0000000000004457.
Sánchez-Álvarez et al., Status of SARS-CoV-2 infection in patients on renal replacement therapy. Report of the COVID-19 Registry of the Spanish Society of Nephrology (SEN), Nefrología, doi:10.1016/j.nefroe.2020.04.002.
Mallat et al., Hydroxychloroquine is associated with slower viral clearance in clinical COVID-19 patients with mild to moderate disease: A retrospective study, Medicine (Baltimore), doi:10.1097/MD.0000000000023720.
Membrillo de Novales et al., Early Hydroxychloroquine Is Associated with an Increase of Survival in COVID-19 Patients: An Observational Study, Preprints, doi:10.20944/preprints202005.0057.v1.
Geleris et al., Observational Study of Hydroxychloroquine in Hospitalized Patients with Covid-19, NEJM, May 7, 2020, doi:10.1056/NEJMoa2012410.
Alberici et al., A report from the Brescia Renal COVID Task Force on the clinical characteristics and short-term outcome of hemodialysis patients with SARS-CoV-2 infection, Kidney Int., 98:1, 20-26, July 1, 2020, doi:10.1016/j.kint.2020.04.030.
Rosenberg et al., Association of Treatment With Hydroxychloroquine or Azithromycin With In-Hospital Mortality in Patients With COVID-19 in New York State, JAMA, May 11, 2020, doi:10.1001/jama.2020.8630.
Shabrawishi et al., Negative nasopharyngeal SARS-CoV-2 PCR conversion in response to different therapeutic interventions, medRxix, doi:10.1101/2020.05.08.20095679.
Mahévas et al., Clinical efficacy of hydroxychloroquine in patients with covid-19 pneumonia who require oxygen: observational comparative study using routine care data, BMJ 2020, doi:10.1136/bmj.m1844.
Yu (B) et al., Low Dose of Hydroxychloroquine Reduces Fatality of Critically Ill Patients With COVID-19, Science China Life Sciences, 2020 May 15, 1-7, doi:10.1007/s11427-020-1732-2.
Kim et al., Treatment Response to Hydroxychloroquine, Lopinavir/Ritonavir, and Antibiotics for Moderate COVID 19: A First Report on the Pharmacological Outcomes from South Korea, medRxiv, doi:10.1101/2020.05.13.20094193.
Singh et al., Outcomes of Hydroxychloroquine Treatment Among Hospitalized COVID-19 Patients in the United States- Real-World Evidence From a Federated Electronic Medical Record Network, medRxiv, doi:10.1101/2020.05.12.20099028.
Luo et al., Metformin Treatment Was Associated with Decreased Mortality in COVID-19 Patients with Diabetes in a Retrospective Analysis, The American Journal of Tropical Medicine and Hygiene, doi:10.4269/ajtmh.20-0375.
Hraiech et al., Lack of viral clearance by the combination of hydroxychloroquine and azithromycin or lopinavir and ritonavir in SARS-CoV-2-related acute respiratory distress syndrome, Ann. Intensive Care, doi:10.1186/s13613-020-00678-4.
Ip (B) et al., Hydroxychloroquine and Tocilizumab Therapy in COVID-19 Patients - An Observational Study, PLoS ONE, doi:10.1371/journal.pone.0237693.
Goldman et al., Remdesivir for 5 or 10 Days in Patients with Severe Covid-19, NEJM, doi:10.1056/NEJMoa2015301.
Huang (B) et al., Preliminary evidence from a multicenter prospective observational study of the safety and efficacy of chloroquine for the treatment of COVID-19, National Science Review, doi:10.1093/nsr/nwaa113.
Kuderer et al., Clinical impact of COVID-19 on patients with cancer (CCC19): a cohort study, Lancet, June 20, 2020, doi:10.1016/S0140-6736(20)31187-9.
Rogado et al., Covid-19 and lung cancer: A greater fatality rate?, Lung Cancer, doi:10.1016/j.lungcan.2020.05.034.
RECOVERY Collaborative Group, Effect of Hydroxychloroquine in Hospitalized Patients with COVID-19: Preliminary results from a multi-centre, randomized, controlled trial, NEJM, doi:10.1056/NEJMoa2022926.
Wang et al., Comorbidity and Sociodemographic determinants in COVID-19 Mortality in an US Urban Healthcare System, medRxiv, doi:10.1101/2020.06.11.20128926.
Luo (B) et al., COVID-19 in patients with lung cancer, Annals of Oncology, 31:10, 1386-1396, doi:10.1016/j.annonc.2020.06.007.
Paccoud et al., Compassionate use of hydroxychloroquine in clinical practice for patients with mild to severe Covid-19 in a French university hospital, Clinical Infectious Diseases, doi:10.1093/cid/ciaa791.
Sbidian et al., Hydroxychloroquine with or without azithromycin and in-hospital mortality or discharge in patients hospitalized for COVID-19 infection: a cohort study of 4,642 in-patients in France, medRxiv, doi:10.1101/2020.06.16.20132597.
Faíco-Filho et al., No benefit of hydroxychloroquine on SARS-CoV-2 viral load reduction in non-critical hospitalized patients with COVID-19, Braz J Microbiol, doi:10.1007/s42770-020-00395-x.
Chen (C) et al., Efficacy and safety of chloroquine or hydroxychloroquine in moderate type of COVID-19: a prospective open-label randomized controlled study, medRxiv, doi:10.1101/2020.06.19.20136093.
Fontana et al., SARS-CoV-2 infection in dialysis patients in northern Italy: a single-centre experience, Clinical Kidney Journal, 13:3, 334–339, doi:10.1093/ckj/sfaa084.
Bousquet et al., ADL-dependency, D-Dimers, LDH and absence of anticoagulation are independently associated with one-month mortality in older inpatients with Covid-19, Aging, 12:12, 11306-11313, doi:10.18632/aging.103583.
Lagier et al., Outcomes of 3,737 COVID-19 patients treated with hydroxychloroquine/azithromycin and other regimens in Marseille, France: A retrospective analysis, Travel Medicine and Infectious Disease, doi:10.1016/j.tmaid.2020.101791.
Sosa-García et al., Experience in the management of severe COVID-19 patients in an intensive care unit, Cir Cir. 2020, 88:5, 569-575, doi:10.24875/CIRU.20000675.
Komissarov et al., Hydroxychloroquine has no effect on SARS-CoV-2 load in nasopharynx of patients with mild form of COVID-19, medRxiv, doi:10.1101/2020.06.30.20143289.
Mikami et al., Risk Factors for Mortality in Patients with COVID-19 in New York City, J. Gen. Intern. Med., doi:10.1007/s11606-020-05983-z.
Martinez-Lopez et al., Multiple Myeloma and SARS-CoV-2 Infection: Clinical Characteristics and Prognostic Factors of Inpatient Mortality, Blood Cancer Journal, doi:10.1038/s41408-020-00372-5.
Arshad et al., Treatment with Hydroxychloroquine, Azithromycin, and Combination in Patients Hospitalized with COVID-19, International Journal of Infectious Diseases, doi:10.1016/j.ijid.2020.06.099.
An et al., Treatment Response to Hydroxychloroquine and Antibiotics for mild to moderate COVID-19: a retrospective cohort study from South Korea, medRxiv, doi:10.1101/2020.07.04.20146548.
Rivera-Izquierdo et al., Agentes terapéuticos utilizados en 238 pacientes hospitalizados por COVID-19 y su relación con la mortalidad, Medicina Clínica, doi:10.1016/j.medcli.2020.06.025.
Chen (D) et al., A Multicenter, randomized, open-label, controlled trial to evaluate the efficacy and tolerability of hydroxychloroquine and a retrospective study in adult patients with mild to moderate Coronavirus disease 2019 (COVID-19), PLoS ONE, doi:10.1371/journal.pone.0242763.
Chen (E) et al., A Multicenter, randomized, open-label, controlled trial to evaluate the efficacy and tolerability of hydroxychloroquine and a retrospective study in adult patients with mild to moderate Coronavirus disease 2019 (COVID-19), PLoS ONE, doi:10.1371/journal.pone.0242763.
Cravedi et al., COVID-19 and kidney transplantation: Results from the TANGO International Transplant Consortium, American Journal of Transplantation, doi:10.1111/ajt.16185.
Lecronier et al., Comparison of hydroxychloroquine, lopinavir/ritonavir, and standard of care in critically ill patients with SARS-CoV-2 pneumonia: an opportunistic retrospective analysis, Critical Care, 24:418, 2020, doi:10.1186/s13054-020-03117-9.
Trullàs et al., High in-hospital mortality due to COVID-19 in a community hospital in Spain: a prospective observational study, Research Square, doi:10.21203/rs.3.rs-39421/v1.
Gupta et al., Factors Associated With Death in Critically Ill Patients With Coronavirus Disease 2019 in the US, JAMA Intern. Med., doi:10.1001/jamainternmed.2020.3596.
Lyngbakken et al., A pragmatic randomized controlled trial reports lack of efficacy of hydroxychloroquine on coronavirus disease 2019 viral kinetics, Nature Communications, doi:10.1038/s41467-020-19056-6.
McGrail et al., COVID-19 Case Series at UnityPoint Health St. Luke’s Hospital in Cedar Rapids, IA, medRxiv, doi:10.1101/2020.07.17.20156521.
Krishnan et al., Clinical comorbidities, characteristics, and outcomes of mechanically ventilated patients in the State of Michigan with SARS-CoV-2 pneumonia, Journal of Clinical Anesthesia, doi:10.1016/j.jclinane.2020.110005.
Bernaola et al., Observational Study of the Efficiency of Treatments in Patients Hospitalized with Covid-19 in Madrid, medRxiv, doi:10.1101/2020.07.17.20155960.
Kelly et al., Clinical outcomes and adverse events in patients hospitalised with COVID-19, treated with off-label hydroxychloroquine and azithromycin, British Journal of Clinical Pharmacology, doi:10.1111/bcp.14482.
Rivera et al., Utilization of COVID-19 Treatments and Clinical Outcomes among Patients with Cancer: A COVID-19 and Cancer Consortium (CCC19) Cohort Study, Cancer Discovery, doi:10.1158/2159-8290.CD-20-0941.
Cavalcanti et al., Hydroxychloroquine with or without Azithromycin in Mild-to-Moderate Covid-19, NEJM, doi:10.1056/NEJMoa2019014.
Santos et al., Determinants of COVID-19 disease severity in patients with underlying rheumatic disease, Clinical Rheumatology, doi:10.1007/s10067-020-05301-2.
Novartis, Hydroxychloroquine Monotherapy and in Combination With Azithromycin in Patients With Moderate and Severe COVID-19 Disease, NCT04358081, clinicaltrials.gov/study/NCT04358081.
D'Arminio Monforte et al., Effectiveness of Hydroxychloroquine in COVID-19 disease: A done and dusted situation?, International Journal of Infectious Diseases, doi:10.1016/j.ijid.2020.07.056.
Davido et al., Impact of medical care including anti-infective agents use on the prognosis of COVID-19 hospitalized patients over time, International Journal of Antimicrobial Agents, 2020, doi:10.1016/j.ijantimicag.2020.106129.
Yu (C) et al., Beneficial effects exerted by hydroxychloroquine in treating COVID-19 patients via protecting multiple organs, Science China Life Sciences, 2020 Aug 3, doi:10.1007/s11427-020-1782-1.
Berenguer et al., Characteristics and predictors of death among 4035 consecutively hospitalized patients with COVID-19 in Spain, Clinical Microbiology and Infection, doi:10.1016/j.cmi.2020.07.024.
Kamran et al., Clearing the fog: Is HCQ effective in reducing COVID-19 progression: A randomized controlled trial, medRxiv, doi:10.1101/2020.07.30.20165365.
Kalligeros et al., Hydroxychloroquine use in hospitalised patients with COVID-19: An observational matched cohort study, Journal of Global Antimicrobial Resistance, doi:10.1016/j.jgar.2020.07.018.
Saleemi et al., Time to negative PCR from symptom onset in COVID-19 patients on Hydroxychloroquine and Azithromycin - A real world experience, medRxiv, doi:10.1101/2020.08.05.20151027.
Pablos et al., Clinical outcomes of hospitalised patients with COVID-19 and chronic inflammatory and autoimmune rheumatic diseases: a multicentric matched cohort study, Annals of the Rheumatic Diseases, doi:10.1136/annrheumdis-2020-218296.
Roomi et al., Efficacy of hydroxychloroquine and tocilizumab in patients with COVID-19: A single-center retrospective chart review, J. Medical Internet Research, doi:10.2196/21758.
Peters et al., Outcomes of Persons With COVID-19 in Hospitals With and Without Standard Treatment With (Hydroxy)chloroquine, Clinical Microbiology and Infection, doi:10.1016/j.cmi.2020.10.004.
Pinato et al., Clinical portrait of the SARS-CoV-2 epidemic in European cancer patients, Cancer Discovery, doi:10.1158/2159-8290.CD-20-0773.
Dubernet et al., A comprehensive strategy for the early treatment of COVID-19 with azithromycin/hydroxychloroquine and/or corticosteroids: results of a retrospective observational study in the French overseas department of Reunion Island, Journal of Global Antimicrobial Resistance, doi:10.1016/j.jgar.2020.08.001.
Gonzalez et al., The Prognostic Value of Eosinophil Recovery in COVID-19: A Multicentre, Retrospective Cohort Study on Patients Hospitalised in Spanish Hospitals, medRxiv, doi:10.1101/2020.08.18.20172874.
Pasquini et al., Effectiveness of remdesivir in patients with COVID-19 under mechanical ventilation in an Italian ICU, Journal of Antimicrobial Chemotherapy, doi:10.1093/jac/dkaa321.
Catteau et al., Low-dose Hydroxychloroquine Therapy and Mortality in Hospitalized Patients with COVID-19: A Nationwide Observational Study of 8075 Participants, International Journal of Antimicrobial Agents, doi:10.1016/j.ijantimicag.2020.106144.
Di Castelnuovo et al., Use of hydroxychloroquine in hospitalised COVID-19 patients is associated with reduced mortality: Findings from the observational multicentre Italian CORIST study, European Journal of Internal Medicine, doi:10.1016/j.ejim.2020.08.019.
Fried et al., Patient Characteristics and Outcomes of 11,721 Patients with COVID19 Hospitalized Across the United States, Clinical Infectious Disease, doi:10.1093/cid/ciaa1268.
Albani et al., Impact of Azithromycin and/or Hydroxychloroquine on Hospital Mortality in COVID-19, J, Clinical Medicine, doi:10.3390/jcm9092800.
Synolaki et al., The Activin/Follistatin-axis is severely deregulated in COVID-19 and independently associated with in-hospital mortality, medRxiv, doi:10.1101/2020.09.05.20184655.
Alamdari et al., Mortality Risk Factors among Hospitalized COVID-19 Patients in a Major Referral Center in Iran, The Tohoku Journal of Experimental Medicine, doi:10.1620/tjem.252.73.
Heberto et al., Implications of myocardial injury in Mexican hospitalized patients with coronavirus disease 2019 (COVID-19), IJC Heart & Vasculature, doi:10.1016/j.ijcha.2020.100638.
Lauriola et al., Effect of combination therapy of hydroxychloroquine and azithromycin on mortality in COVID-19 patients, Clinical and Translational Science, doi:10.1111/cts.12860.
Ashinyo et al., Clinical characteristics, treatment regimen and duration of hospitalization among COVID-19 patients in Ghana: a retrospective cohort study, Pan African Medical Journal, 37:1, doi:10.11604/pamj.supp.2020.37.1.25718.
Serrano et al., COVID-19 and lung cancer: What do we know?, Ann. Oncol., 2020, Sep, 31, S1026, doi:10.1016/j.annonc.2020.08.1830.
Ulrich et al., Treating Covid-19 With Hydroxychloroquine (TEACH): A Multicenter, Double-Blind, Randomized Controlled Trial in Hospitalized Patients, Open Forum Infectious Diseases, doi:10.1093/ofid/ofaa446.
Shoaibi et al., Comparative Effectiveness of Famotidine in Hospitalized COVID-19 Patients, medRxiv, doi:10.1101/2020.09.23.20199463.
Lammers et al., Early hydroxychloroquine but not chloroquine use reduces ICU admission in COVID-19 patients, International Journal of Infectious Diseases, doi:10.1016/j.ijid.2020.09.1460.
Ayerbe et al., The association of treatment with hydroxychloroquine and hospital mortality in COVID-19 patients, Internal and Emergency Medicine, doi:10.1007/s11739-020-02505-x.
Almazrou et al., Comparing the impact of Hydroxychloroquine based regimens and standard treatment on COVID-19 patient outcomes: A retrospective cohort study, Saudi Pharmaceutical Journal, doi:10.1016/j.jsps.2020.09.019.
Nachega et al., Clinical Characteristics and Outcomes of Patients Hospitalized for COVID-19 in Africa: Early Insights from the Democratic Republic of the Congo, The American Journal of Tropical Medicine and Hygiene, doi:10.4269/ajtmh.20-1240.
Ader et al., An open-label randomized, controlled trial of the effect of lopinavir/ritonavir, lopinavir/ritonavir plus IFN-beta-1a and hydroxychloroquine in hospitalized patients with COVID-19 - Final results from the DisCoVeRy trial, medRxiv, doi:10.1101/2022.02.16.22271064.
Soto-Becerra (B) et al., Real-World Effectiveness of hydroxychloroquine, azithromycin, and ivermectin among hospitalized COVID-19 patients: Results of a target trial emulation using observational data from a nationwide Healthcare System in Peru, medRxiv, doi:10.1101/2020.10.06.20208066.
Aparisi et al., Low-density lipoprotein cholesterol levels are associated with poor clinical outcomes in COVID-19, medRxiv, doi:10.1101/2020.10.06.20207092.
Annie et al., Hydroxychloroquine in hospitalized COVID-19 patients: Real world experience assessing mortality, Pharmacotherapy, doi:10.1002/phar.2467.
SOLIDARITY Trial Consortium, Repurposed antiviral drugs for COVID-19; interim WHO SOLIDARITY trial results, NEJM, doi:10.1056/NEJMoa2023184.
Guisado-Vasco (B) et al., Clinical characteristics and outcomes among hospitalized adults with severe COVID-19 admitted to a tertiary medical center and receiving antiviral, antimalarials, glucocorticoids, or immunomodulation with tocilizumab or cyclosporine: A retrospective observational study (COQUIMA cohort), eClinicalMedicine, doi:10.1016/j.eclinm.2020.100591.
Solh et al., Clinical course and outcome of COVID-19 acute respiratory distress syndrome: data from a national repository, medRxiv, doi:10.1101/2020.10.16.20214130.
Ñamendys-Silva et al., Outcomes of patients with COVID-19 in the Intensive Care Unit in Mexico: A multicenter observational study, Heart & Lung, doi:10.1016/j.hrtlng.2020.10.013.
Dubee et al., Hydroxychloroquine in mild-to-moderate COVID-19: a placebo-controlled double blind trial, Clinical Microbiology and Infection, doi:10.1016/j.cmi.2021.03.005.
Lano et al., Risk factors for severity of COVID-19 in chronic dialysis patients from a multicentre French cohort, Clinical Kidney Journal, 13:5, October 2020, 878–888, doi:10.1093/ckj/sfaa199.
Coll et al., Covid-19 in transplant recipients: the spanish experience, American Journal of Transplantation, doi:10.1111/ajt.16369.
Frontera et al., Treatment with Zinc is Associated with Reduced In-Hospital Mortality Among COVID-19 Patients: A Multi-Center Cohort Study, Research Square, doi:10.21203/rs.3.rs-94509/v1.
Choi et al., Comparison of antiviral effect for mild-to-moderate COVID-19 cases between lopinavir/ritonavir versus hydroxychloroquine: A nationwide propensity score-matched cohort study, International Journal of Infectious Diseases, doi:10.1016/j.ijid.2020.10.062.
Tehrani et al., Risk factors for mortality in adult COVID-19 patients: frailty predicts fatal outcome in older patients, International Journal of Infectious Diseases, doi:10.1016/j.ijid.2020.10.071.
Niwas et al., Clinical outcome, viral response and safety profile of chloroquine in COVID-19 patients — initial experience, Advances in Respiratory Medicine, doi:10.5603/ARM.a2020.0139.
López et al., Telemedicine follow-ups for COVID-19: experience in a tertiary hospital, Annals of Pediatrics, doi:10.1016/j.anpedi.2020.10.017.
Salazar et al., Significantly Decreased Mortality in a Large Cohort of Coronavirus Disease 2019 (COVID-19) Patients Transfused Early with Convalescent Plasma Containing High-Titer Anti–Severe Acute Respiratory Syndrome Coronavirus 2 (SARS-CoV-2) Spike Protein IgG, The American Journal of Pathology, doi:10.1016/j.ajpath.2020.10.008.
Maldonado et al., COVID-19 incidence and outcomes in a home dialysis unit in Madrid (Spain) at the height of the pandemic, Nefrología, doi:10.1016/j.nefro.2020.09.002.
Núñez-Gil et al., Mortality risk assessment in Spain and Italy, insights of the HOPE COVID-19 registry, Internal and Emergency Medicine, doi:10.1007/s11739-020-02543-5.
Self et al., Effect of Hydroxychloroquine on Clinical Status at 14 Days in Hospitalized Patients With COVID-19: A Randomized Clinical Trial, JAMA, doi:10.1001/jama.2020.22240.
Rodriguez et al., Severe infection due to the SARS-CoV-2 coronavirus: Experience of a tertiary hospital with COVID-19 patients during the 2020 pandemic, Medicina Intensiva, doi:10.1016/j.medine.2020.05.005.
Águila-Gordo et al., Mortality and associated prognostic factors in elderly and very elderly hospitalized patients with respiratory disease COVID-19, Revista Española de Geriatría y Gerontología, doi:10.1016/j.regg.2020.09.006.
Sheshah et al., Prevalence of Diabetes, Management and Outcomes among Covid-19 Adult Patients Admitted in a Specialized Tertiary Hospital in Riyadh, Saudi Arabia, Diabetes Research and Clinical Practice, doi:10.1016/j.diabres.2020.108538.
Hofmann-Winkler et al., Camostat Mesylate May Reduce Severity of Coronavirus Disease 2019 Sepsis: A First Observation, Critical Care Explorations, doi:10.1097/CCE.0000000000000284.
Boari et al., Prognostic factors and predictors of outcome in patients with COVID-19 and related pneumonia: a retrospective cohort study, Bioscience Reports, doi:10.1042/BSR20203455.
Budhiraja (B) et al., Clinical Profile of First 1000 COVID-19 Cases Admitted at Tertiary Care Hospitals and the Correlates of their Mortality: An Indian Experience, medRxiv, doi:10.1101/2020.11.16.20232223.
Falcone et al., Role of low-molecular weight heparin in hospitalized patients with SARS-CoV-2 pneumonia: a prospective observational study, Open Forum Infectious Diseases, doi:10.1093/ofid/ofaa563.
Qin et al., Low molecular weight heparin and 28-day mortality among patients with coronavirus disease 2019: A cohort study in the early epidemic era, Thrombosis Research, doi:10.1016/j.thromres.2020.11.020.
Burdick et al., Is Machine Learning a Better Way to IdentifyCOVID-19 Patients Who Might Benefit fromHydroxychloroquineTreatment?—The IDENTIFY Trial, Journal of Clinical Medicine, doi:10.3390/jcm9123834.
van Halem et al., Risk factors for mortality in hospitalized patients with COVID-19 at the start of the pandemic in Belgium: a retrospective cohort study, BMC Infectious Diseases, doi:10.1186/s12879-020-05605-3.
Rodriguez-Gonzalez et al., COVID-19 in hospitalized patients in Spain: a cohort study in Madrid, International Journal of Antimicrobial Agents, doi:10.1016/j.ijantimicag.2020.106249.
Lambermont et al., Predictors of Mortality and Effect of Drug Therapies in Mechanically Ventilated Patients With Coronavirus Disease 2019: A Multicenter Cohort Study, Critical Care Explorations, doi:10.1097/CCE.0000000000000305.
Abdulrahman et al., The efficacy and safety of hydroxychloroquine in COVID19 patients : a multicenter national retrospective cohort, medRxiv, doi:10.1101/2020.11.25.20234914.
Aboulenain et al., The Effect of Hydroxychloroquine on In-Hospital Mortality in COVID-19, HCA Healthcare Journal of Medicine, doi:10.36518/2689-0216.1169.
Capsoni et al., CPAP Treatment In COVID-19 Patients: A Retrospective Observational Study In The Emergency Department, Research Square, doi:10.21203/rs.3.rs-113418/v1.
Peng et al., Early versus late acute kidney injury among patients with COVID-19—a multicenter study from Wuhan, China, Nephrology Dialysis Transplantation, doi:10.1093/ndt/gfaa288.
Modrák et al., Detailed disease progression of 213 patients hospitalized with Covid-19 in the Czech Republic: An exploratory analysis, medRxiv, doi:10.1101/2020.12.03.20239863.
Ozturk et al., Mortality analysis of COVID-19 infection in chronic kidney disease, haemodialysis and renal transplant patients compared with patients without kidney disease: a nationwide analysis from Turkey, Nephrology Dialysis Transplantation, doi:10.1093/ndt/gfaa271.
Guglielmetti et al., Severe COVID-19 pneumonia in Piacenza, Italy – a cohort study of the first pandemic wave, Journal of Infection and Public Health, doi:10.1016/j.jiph.2020.11.012.
Johnston et al., Hydroxychloroquine with or Without Azithromycin for Treatment of Early SARS-CoV-2 Infection Among High-Risk Outpatient Adults: A Randomized Clinical Trial, eClinicalMedicine, doi:10.1016/j.eclinm.2021.100773.
Alqassieh et al., Clinical characteristics and predictors of the duration of hospital stay in COVID-19 patients in Jordan, F1000Research, doi:10.12688/f1000research.27419.1.
Rosenthal et al., Risk Factors Associated With In-Hospital Mortality in a US National Sample of Patients With COVID-19, JAMA Network Open, doi:10.1001/jamanetworkopen.2020.29058.
Bielza et al., Clinical characteristics, frailty and mortality of residents with COVID-19 in nursing homes of a region of Madrid, Journal of the American Medical Directors Association, doi:10.1016/j.jamda.2020.12.003.
Tan et al., A retrospective comparison of drugs against COVID-19, Virus Research, doi:10.1016/j.virusres.2020.198262.
Naseem et al., Predicting mortality in SARS-COV-2 (COVID-19) positive patients in the inpatient setting using a Novel Deep Neural Network, medRxiv, doi:10.1101/2020.12.13.20247254.
Orioli et al., Clinical characteristics and short-term prognosis of in-patients with diabetes and COVID-19: A retrospective study from an academic center in Belgium, Diabetes & Metabolic Syndrome: Clinical Research & Reviews, doi:10.1016/j.dsx.2020.12.020.
De Luna et al., Clinical and Demographic Characteristics of COVID-19 Patients Admitted in a Tertiary Care Hospital in the Dominican Republic, medRxiv, doi:10.1101/2020.12.11.20247437.
Signes-Costa et al., Prevalence and 30-day mortality in hospitalized patients with COVID-19 and prior lung diseases, Archivos de Bronconeumología, doi:10.1016/j.arbres.2020.11.012.
Matangila et al., Clinical characteristics of COVID-19 patients hospitalized at Clinique Ngaliema, a public hospital in Kinshasa, in the Democratic Republic of Congo: A retrospective cohort study, PLoS ONE, doi:10.1371/journal.pone.0244272.
Cangiano et al., Mortality in an Italian nursing home during COVID-19 pandemic: correlation with gender, age, ADL, vitamin D supplementation, and limitations of the diagnostic tests, Aging, doi:10.18632/aging.202307.
Taccone et al., The role of organizational characteristics on the outcome of COVID-19 patients admitted to the ICU in Belgium, The Lancet Regional Health - Europe, doi:10.1016/j.lanepe.2020.100019.
Chari et al., Clinical features associated with COVID-19 outcome in multiple myeloma: first results from the International Myeloma Society data set, Blood, doi:10.1182/blood.2020008150.
Güner et al., Comparing ICU Admission Rates of Mild/Moderate COVID-19 Patients Treated with Hydroxychloroquine, Favipiravir, and Hydroxychloroquine plus Favipiravir, Journal of Infection and Public Health, doi:10.1016/j.jiph.2020.12.017.
Vernaz et al., Early experimental COVID-19 therapies: associations with length of hospital stay, mortality and related costs, Swiss Medical Weekly, doi:10.4414/smw.2020.20446.
Texeira et al., Characteristics and outcomes of COVID-19 patients admitted to a regional health system in the southeast, Open Forum Infectious Diseases, doi:10.1093/ofid/ofaa439.560.
Psevdos et al., Corona Virus Disease-19 (COVID-19) in a Veterans Affairs Hospital at Suffolk County, Long Island, New York, Open Forum Infectious Diseases, doi:10.1093/ofid/ofaa439.721.
Mahale et al., A Retrospective Observational Study of Hypoxic COVID-19 Patients Treated with Immunomodulatory Drugs in a Tertiary Care Hospital, Indian Journal of Critical Care Medicine, doi:10.5005/jp-journals-10071-23599.
Sands et al., No clinical benefit in mortality associated with hydroxychloroquine treatment in patients with COVID-19, International Journal of Infectious Diseases, doi:10.1016/j.ijid.2020.12.060.
Lotfy et al., Use of Hydroxychloroquine in Patients with COVID-19: A Retrospective Observational Study, Turk. Thorac. J., doi:10.5152/TurkThoracJ.2021.20180.
Sarfaraz et al., Determinants of in-hospital mortality in COVID-19; a prospective cohort study from Pakistan, medRxiv, doi:10.1101/2020.12.28.20248920.
Yegerov et al., Epidemiological and Clinical Characteristics, and Virologic Features of COVID-19 Patients in Kazakhstan: a Nation-Wide, Retrospective, Cohort Study, medRxiv, doi:10.1101/2021.01.06.20249091.
Li et al., Treatment of COVID-19 patients with hydroxychloroquine or chloroquine: A retrospective analysis, Research Square, doi:10.21203/rs.3.rs-119202/v1.
Li (B) et al., Evaluation of the efficacy and safety of hydroxychloroquine in comparison with chloroquine in moderate and severe patients with COVID-19, Science China Life Sciences, doi:10.1007/s11427-020-1871-4.
Di Castelnuovo (B) et al., Disentangling the Association of Hydroxychloroquine Treatment with Mortality in Covid-19 Hospitalized Patients through Hierarchical Clustering, Journal of Healthcare Engineering, doi:10.1155/2021/5556207.
Roig et al., Clinical and pharmacological data in COVID-19 hospitalized nonagenarian patients, Revista Espanola de Quimioterapia, doi:10.37201/req/130.2020.
Ubaldo et al., COVID-19: A Single-Center ICU Experience of the First Wave in the Philippines, Critical Care Research and Practice, doi:10.1155/2021/7510306.
Ouedraogo et al., Factors associated with the occurrence of acute respiratory distress and death in patients with COVID-19 in Burkina Faso, Revue des Maladies Respiratoires, doi:10.1016/j.rmr.2021.02.001.
Hernandez-Cardenas et al., Hydroxychloroquine for the treatment of severe respiratory infection by COVID-19: a randomized controlled trial, medRxiv, doi:10.1101/2021.02.01.21250371.
Purwati et al., A Randomized, Double-Blind, Multicenter Clinical Study Comparing the Efficacy and Safety of a Drug Combination of Lopinavir/Ritonavir-Azithromycin, Lopinavir/Ritonavir-Doxycycline, and Azithromycin-Hydroxychloroquine for Patients Diagnosed with Mild to Moderate COVID-19 Infections, Biochemistry Research International, doi:10.1155/2021/6685921.
Lora-Tamayo et al., Early Lopinavir/ritonavir does not reduce mortality in COVID-19 patients: results of a large multicenter study, Journal of Infection, doi:10.1016/j.jinf.2021.02.011.
Baguiya et al., Effect of Hydroxychloroquine or Chloroquine and Azithromycin on COVID-19 Patients' Recovery and Mortality: Evidence from a Hospital Based Retrospective Cohort Study Conducted in Burkina Faso, Journal of Infectious Diseases and Epidemiology, doi:10.23937/2474-3658/1510192.
Awad et al., Impact of hydroxychloroquine on disease progression and ICU admissions in patients with SARS-CoV-2 infection, American Journal of Health-System Pharmacy, doi:10.1093/ajhp/zxab056.
Lamback et al., Hydroxychloroquine with azithromycin in patients hospitalized for mild and moderate COVID-19, The Brazilian Journal of Infectious Diseases, doi:10.1016/j.bjid.2021.101549.
Beltran Gonzalez (B) et al., Efficacy and Safety of Ivermectin and Hydroxychloroquine in Patients with Severe COVID-19: A Randomized Controlled Trial, Infectious Disease Reports, doi:10.3390/idr14020020.
Rubio-Sánchez et al., Prognostic factors for the severity of SARS-CoV-2 infection, Advances in Laboratory Medicine / Avances en Medicina de Laboratorio, doi:10.1515/almed-2021-0017.
Salvador et al., Clinical Features and Prognostic Factors of 245 Portuguese Patients Hospitalized With COVID-19, Cureus, doi:10.7759/cureus.13687.
Martin-Vicente et al., Absent or insufficient anti-SARS-CoV-2 S antibodies at ICU admission are associated to higher viral loads in plasma, antigenemia and mortality in COVID-19 patients, medRxiv, doi:10.1101/2021.03.08.21253121.
Stewart et al., COVID-19 Evidence Accelerator: A parallel analysis to describe the use of Hydroxychloroquine with or without Azithromycin among hospitalized COVID-19 patients, PLoS ONE, doi:10.1371/journal.pone.0248128.
Barry et al., Clinical Characteristics and Outcomes of Hospitalized COVID-19 Patients in a MERS-CoV Referral Hospital during the Peak of the Pandemic, International Journal of Infectious Diseases, doi:10.1016/j.ijid.2021.03.058.
Alghamdi et al., Clinical Efficacy of Hydroxychloroquine in Patients with COVID-19: Findings from an Observational Comparative Study in Saudi Arabia, Antibiotics, doi:10.3390/antibiotics10040365.
Mulhem et al., 3219 hospitalised patients with COVID-19 in Southeast Michigan: a retrospective case cohort study, BMJ Open, doi:10.1136/bmjopen-2020-042042.
Gadhiya et al., Clinical characteristics of hospitalised patients with COVID-19 and the impact on mortality: a single-network, retrospective cohort study from Pennsylvania state, BMJ Open, doi:10.1136/bmjopen-2020-042549.
Reis (B) et al., Effect of Early Treatment With Hydroxychloroquine or Lopinavir and Ritonavir on Risk of Hospitalization Among Patients With COVID-19 The TOGETHER Randomized Clinical Trial, JAMA Network Open, doi:10.1001/jamanetworkopen.2021.6468.
Mohandas et al., Clinical review of COVID-19 patients presenting to a quaternary care private hospital in South India: A retrospective study, Clinical Epidemiology and Global Health, doi:10.1016/j.cegh.2021.100751.
Réa-Neto et al., An open-label randomized controlled trial evaluating the efficacy of chloroquine/hydroxychloroquine in severe COVID-19 patients, Scientific Reports, doi:10.1038/s41598-021-88509-9.
Kokturk et al., The predictors of COVID-19 mortality in a nationwide cohort of Turkish patients, Respiratory Medicine, doi:10.1016/j.rmed.2021.106433.
Haji Aghajani et al., Decreased in-hospital mortality associated with aspirin administration in hospitalized patients due to severe COVID-19, Journal of Medical Virology, doi:10.1002/jmv.27053.
Bosaeed et al., Favipiravir and Hydroxychloroquine Combination Therapy in Patients with Moderate to Severe COVID19 (FACCT Trial): An Open-Label, Multicenter, Randomized, Controlled Trial, Infect. Dis. Ther., doi:10.1007/s40121-021-00496-6.
Çiyiltepe et al., The Effect of Pre-admission Hydroxychloroquine Treatment on COVID-19-Related Intensive Care Follow-up in Geriatric Patients, South. Clin. Ist. Euras., doi:10.14744/scie.2021.89847.
De Rosa et al., Risk Factors for Mortality in COVID-19 Hospitalized Patients in Piedmont, Italy: Results from the Multicenter, Regional, CORACLE Registry, Journal of Clinical Medicine, doi:10.3390/jcm10091951.
Sammartino et al., Predictors for inpatient mortality during the first wave of the SARS-CoV-2 pandemic: A retrospective analysis, PLOS One, doi:10.1371/journal.pone.0251262.
Smith (B) et al., Observational Study on 255 Mechanically Ventilated Covid Patients at the Beginning of the USA Pandemic, medRxiv, doi:10.1101/2021.05.28.21258012.
Ramírez-García et al., Hydroxychloroquine and Tocilizumab in the Treatment of COVID-19: A Longitudinal Observational Study, Archivos de Medicina Universitaria, 3:1, digibug.ugr.es/handle/10481/69170.
Sivapalan et al., Azithromycin and hydroxychloroquine in hospitalised patients with confirmed COVID-19–a randomised double-blinded placebo-controlled trial, European Respiratory Journal, doi:10.1183/13993003.00752-2021.
Byakika-Kibwika et al., Safety and Efficacy of Hydroxychloroquine for Treatment of Non-Severe COVID-19 in Adults in Uganda: A Randomized Open Label Phase II Clinical Trial, Research Square, doi:10.21203/rs.3.rs-506195/v1.
Lagier (B) et al., Outcomes of 2,111 COVID-19 hospitalised patients treated with 2 hydroxychloroquine/azithromycin and other regimens in Marseille, France: a 3 monocentric retrospective analysis, Therapeutics and Clinical Risk Management, doi:10.2147/TCRM.S364022.
Singh (B) et al., Safety and efficacy of antiviral therapy alone or in combination in COVID-19 - a randomized controlled trial (SEV COVID Trial), medRxiv, doi:10.1101/2021.06.06.21258091.
Saib et al., Lack of efficacy of hydroxychloroquine and azithromycin in patients hospitalized for COVID-19 pneumonia: A retrospective study, PLOS ONE, doi:10.1371/journal.pone.0252388.
Turrini et al., Clinical Course and Risk Factors for In-Hospital Mortality of 205 Patients with SARS-CoV-2 Pneumonia in Como, Lombardy Region, Italy, Vaccines, doi:10.3390/vaccines9060640.
Schwartz et al., Assessing the efficacy and safety of hydroxychloroquine as outpatient treatment of COVID-19: a randomized controlled trial, CMAJ Open, doi:10.9778/cmajo.20210069.
Gerlovin et al., Pharmacoepidemiology, Machine Learning and COVID-19: An intent-to-treat analysis of hydroxychloroquine, with or without azithromycin, and COVID-19 outcomes amongst hospitalized US Veterans, American Journal of Epidemiology, doi:10.1093/aje/kwab183.
Taieb et al., Hydroxychloroquine and Azithromycin Treatment of Hospitalized Patients Infected with SARS-CoV-2 in Senegal from March to October 2020, Journal of Clinical Medicine, doi:10.3390/jcm10132954.
Jacobs et al., Multi-institutional Analysis of 200 COVID-19 Patients treated with ECMO:Outcomes and Trends, The Annals of Thoracic Surgery, doi:10.1016/j.athoracsur.2021.06.026.
Roger et al., French Multicentre Observational Study on SARS-CoV-2 infections Intensive care initial management: the FRENCH CORONA Study, Anaesthesia Critical Care & Pain Medicine, doi:10.1016/j.accpm.2021.100931.
Tamura et al., Outcome and death risk of diabetes patients with Covid-19 receiving pre-hospital and in-hospital metformin therapies, Diabetology & Metabolic Syndrome, doi:10.1186/s13098-021-00695-8.
Barrat-Due et al., Evaluation of the Effects of Remdesivir and Hydroxychloroquine on Viral Clearance in COVID-19, Annals of Internal Medicine, doi:10.7326/M21-0653.
Alhamlan et al., Epidemiology and Clinical Characteristics in Individuals with Confirmed SARS-CoV-2 Infection During the Early COVID-19 Pandemic in Saudi Arabia, medRxiv, doi:10.1101/2021.07.13.21260428.
Barra et al., COVID-19 in hospitalized patients in 4 hospitals in San Isidro, Buenos Aires, Argentina, medRxiv, doi:10.1101/2021.07.30.21261220.
Alghamdi (B) et al., Clinical characteristics and treatment outcomes of severe (ICU) COVID-19 patients in Saudi Arabia: A single centre study, Saudi Pharmaceutical Journal, doi:10.1016/j.jsps.2021.08.008.
Darcis et al., Long-term clinical follow-up of patients suffering from moderate-to-severe COVID-19 infection: a monocentric prospective observational cohort study, International Journal of Infectious Diseases, doi:10.1016/j.ijid.2021.07.016.
Karruli et al., Multidrug-Resistant Infections and Outcome of Critically Ill Patients with Coronavirus Disease 2019: A Single Center Experience, Microbial Drug Resistance, doi:10.1089/mdr.2020.0489.
Alotaibi et al., Effectiveness and Safety of Favipiravir Compared to Hydroxychloroquine for Management of Covid-19: A Retrospective Study, International Journal of General Medicine, 2021:14, www.dovepress.com/getfile.php?fileID=73585.
Çivriz Bozdağ et al., Clinical Characteristics and Outcome of COVID-19 in Turkish Hematological Malignancy Patients, Turk. J. Haematol., doi:10.4274/tjh.galenos.2021.2021.0287.
Uygen et al., Effect of Hydroxychloroquine Use on the Length Of Hospital Stay in Children Diagnosed With Covid 19, Northern Clinics of Istanbul, doi:10.14744/nci.2021.65471.
Menardi et al., A retrospective analysis on pharmacological approaches to COVID-19 patients in an Italian hub hospital during the early phase of the pandemic, PharmAdvances, doi:10.36118/pharmadvances.2021.15.
Panda et al., Antiviral Combination Clinically Better Than Standard Therapy in Severe but Not in Non-Severe COVID-19, Clinical Pharmacology: Advances and Applications, doi:10.2147/CPAA.S325083.
Babalola (B) et al., A Randomized Controlled Trial of Ivermectin Monotherapy Versus Hydroxychloroquine, Ivermectin, and Azithromycin Combination Therapy in Covid-19 Patients in Nigeria, Journal of Infectious Diseases and Epidemiology, doi:10.23937/2474-3658/1510233.
Guglielmetti (B) et al., Treatment for COVID-19—a cohort study from Northern Italy, Scientific Reports, doi:10.1038/s41598-021-00243-4.
Sarhan et al., Efficacy of the early treatment with tocilizumab-hydroxychloroquine and tocilizumab-remdesivir in severe COVID-19 Patients, Journal of Infection and Public Health, doi:10.1016/j.jiph.2021.10.024.
Cortez et al., Clinical characteristics and outcomes of COVID-19 patients in a tertiary hospital in Baguio City, Philippines, Western Pacific Surveillance and Response Journal, doi:10.5365/wpsar.2021.12.4.852.
Schmidt et al., Association Between Androgen Deprivation Therapy and Mortality Among Patients With Prostate Cancer and COVID-19, JAMA Network Open, doi:10.1001/jamanetworkopen.2021.34330.
Calderón et al., Treatment with hydroxychloroquine vs nitazoxanide in patients with COVID-19: brief report, PAMJ - Clinical Medicine, doi:10.11604/pamj-cm.2021.7.15.30981.
Ferreira (B) et al., Outcomes associated with Hydroxychloroquine and Ivermectin in hospitalized patients with COVID-19: a single-center experience, Revista da Associação Médica Brasileira, doi:10.1590/1806-9282.20210661.
AbdelGhaffar et al., Prediction of mortality in hospitalized Egyptian patients with Coronavirus disease-2019: A multicenter retrospective study, PLOS ONE, doi:10.1371/journal.pone.0262348.
Tu et al., Risk Factors for Severity and Mortality in Adult Patients Confirmed with COVID-19 in Sierra Leone: A Retrospective Study, Infectious Diseases & Immunity, doi:10.1097/ID9.0000000000000037.
Alwafi et al., Negative Nasopharyngeal SARS-CoV-2 PCR Conversion in Response to Different Therapeutic Interventions, Cureus, doi:10.7759/cureus.21442.
Lavilla Olleros et al., Use of glucocorticoids megadoses in SARS-CoV-2 infection in a spanish registry: SEMI-COVID-19, PLOS ONE, doi:10.1371/journal.pone.0261711.
Omma et al., Hydroxychloroquine shortened hospital stay and reduced intensive care unit admissions in hospitalized COVID-19 patients, The Journal of Infection in Developing Countries, doi:10.3855/jidc.14933.
Fernández-Cruz et al., Higher mortality of hospitalized haematologic patients with COVID-19 compared to non-haematologic is driven by thrombotic complications and development of ARDS: An age-matched cohorts study, Clinical Infection in Practice, doi:10.1016/j.clinpr.2022.100137.
Albanghali et al., Clinical Characteristics and Treatment Outcomes of Mild to Moderate Covid-19 Patients in Saudi Arabia: A Single Centre Study, Journal of Infection and Public Health, doi:10.1016/j.jiph.2022.02.001.
Beaumont et al., Factors associated with hospital admission and adverse outcome for COVID-19: role of social factors and medical care, Infectious Diseases Now, doi:10.1016/j.idnow.2022.02.001.
Hall et al., Multi-institutional Analysis of 505 COVID-19 Patients Supported with ECMO: Predictors of Survival, The Annals of Thoracic Surgery, doi:10.1016/j.athoracsur.2022.01.043.
Soto (B) et al., Mortality and associated risk factors in patients hospitalized due to COVID-19 in a Peruvian reference hospital, PLOS ONE, doi:10.1371/journal.pone.0264789.
Tsanovska et al., Hydroxychloroquine (HCQ) treatment for hospitalized patients with COVID-19, Infectious Disorders - Drug Targets, doi:10.2174/1871526522666220303121209.
Azaña Gómez et al., Mortality risk factors in patients with SARS-CoV-2 infection and atrial fibrillation: Data from the SEMI-COVID-19 registry, Medicina Clínica, doi:10.1016/j.medcli.2022.01.008.
Salehi et al., Risk factors of death in mechanically ventilated COVID-19 patients: a retrospective multi-center study, Research Square, doi:10.21203/rs.3.rs-1362678/v1.
Uyaroğlu et al., Comparison of Favipiravir to Hydroxychloroquine Plus Azithromycin in the Treatment of Patients with Non-critical COVID-19: A Single-center, Retrospective, Propensity Score-matched Study, Acta Medica, doi:10.32552/2022.ActaMedica.719.
Ebongue et al., Factors predicting in-hospital all-cause mortality in COVID 19 patients at the Laquintinie Hospital Douala, Cameroon, Travel Medicine and Infectious Disease, doi:10.1016/j.tmaid.2022.102292.
AlQahtani et al., Randomized controlled trial of favipiravir, hydroxychloroquine, and standard care in patients with mild/moderate COVID-19 disease, Scientific Reports, doi:10.1038/s41598-022-08794-w.
Hafez et al., Antiviral Used among Non-Severe COVID-19 Cases in Relation to Time till Viral Clearance: A Retrospective Cohort Study, Antibiotics, doi:10.3390/antibiotics11040498.
Bassets-Bosch et al., Negativización de PCR a SARS-CoV-2 en muestra respiratoria en pacientes con necesidad de asistencia recurrente, Anales de Pediatría, doi:10.1016/j.anpedi.2021.01.006.
Hong (B) et al., Use of combined treatment of 3rd-generation cephalosporin, azithromycin and antiviral agents on moderate SARs-CoV-2 patients in South Korea: A retrospective cohort study, PLOS ONE, doi:10.1371/journal.pone.0267645.
Silva et al., Clinical-Epidemiology Aspect of Inpatients With Moderate or Severe COVID-19 in a Brazilian Macroregion: Disease and Countermeasures, Frontiers in Cellular and Infection Microbiology, doi:10.3389/fcimb.2022.899702.
Osawa et al., Characteristics and risk factors for mortality in critically ill patients with COVID-19 receiving invasive mechanical ventilation: the experience of a private network in Sao Paulo, Brazil, The Journal of Critical Care Medicine, doi:10.2478/jccm-2022-0015.
Malundo et al., Predictors of Mortality among inpatients with COVID-19 Infection in a Tertiary Referral Center in the Philippines, IJID Regions, doi:10.1016/j.ijregi.2022.07.009.
Lyashchenko et al., Systemic Exposure to Hydroxychloroquine and its relationship with outcome in severely ill COVID-19 patients in New York City, British Journal of Clinical Pharmacology, doi:10.1111/bcp.15489.
Bowen et al., Reduction in risk of death among patients admitted with COVID-19 between first and second epidemic waves in New York City, Open Forum Infectious Diseases, doi:10.1093/ofid/ofac436.
Babayigit et al., The association of antiviral drugs with COVID-19 morbidity: The retrospective analysis of a nationwide COVID-19 cohort, Frontiers in Medicine, doi:10.3389/fmed.2022.894126.
Núñez-Gil (B) et al., Hydroxychloroquine and Mortality in SARS-Cov-2 Infection; The HOPE- Covid-19 Registry., Anti-Infective Agents, doi:10.2174/2211352520666220514112951.
Go et al., Hydroxychloroquine, azithromycin and methylprednisolone and in hospital survival in severe COVID-19 pneumonia, Frontiers in Pharmacology, doi:10.3389/fphar.2022.935370.
Assad, H., Pharmacotherapy prescribing pattern and outcome for hospitalized patients with severe and critical COVID-19, Current Issues in Pharmacy and Medical Sciences, doi:10.2478/cipms-2022-0020.
Bubenek-Turconi et al., Clinical characteristics and factors associated with ICU mortality during the first year of the SARS-Cov-2 pandemic in Romania, European Journal of Anaesthesiology, doi:10.1097/EJA.0000000000001776.
Alosaimi et al., Analyzing the Difference in the Length of Stay (LOS) in Moderate to Severe COVID-19 Patients Receiving Hydroxychloroquine or Favipiravir, Pharmaceuticals, doi:10.3390/ph15121456.
Charif et al., Predictive Factors of Death and the Clinical Profile of Hospitalized Covid-19 Patients in Morocco: A One-Year Mixed Cohort Study, Cureus, doi:10.7759/cureus.32462.
Higgins et al., Long-term (180-Day) Outcomes in Critically Ill Patients With COVID-19 in the REMAP-CAP Randomized Clinical Trial, JAMA, doi:10.1001/jama.2022.23257.
Alshamrani et al., Comprehensive evaluation of six interventions for hospitalized patients with COVID-19: A propensity score matching study, Saudi Pharmaceutical Journal, doi:10.1016/j.jsps.2023.02.004.
Delgado et al., Investigational medications in 9,638 hospitalized patients with severe COVID-19: lessons from the “fail-and-learn” strategy during the first two waves of the pandemic in 2020, Research Square, doi:10.21203/rs.3.rs-2596201/v1.
Spivak et al., A Randomized Clinical Trial Testing Hydroxychloroquine for Reduction of SARS-CoV-2 Viral Shedding and Hospitalization in Early Outpatient COVID-19 Infection, Microbiology Spectrum, doi:10.1128/spectrum.04674-22.
Aweimer et al., Mortality rates of severe COVID-19-related respiratory failure with and without extracorporeal membrane oxygenation in the Middle Ruhr Region of Germany, Scientific Reports, doi:10.1038/s41598-023-31944-7.
Ho et al., Hydroxychloroquine for COVID-19: A Single Center, Retrospective Cohort Study, Malaysian Journal of Medicine and Health Sciences, doi:10.47836/mjmhs.19.2.3.
Krishnan (B) et al., Predictors of Mortality among Patients Hospitalized with COVID-19 during the First Wave in India: A Multisite Case-Control Study, The American Journal of Tropical Medicine and Hygiene, doi:10.4269/ajtmh.22-0705.
Said et al., Profiles of Independent-Comorbidity Groups in Senior COVID-19 Patients Reveal Low Fatality Associated with Standard Care and Low-Dose Hydroxychloroquine over Antivirals, Journal of Multidisciplinary Healthcare, doi:10.2147/JMDH.S403700.
AlQadheeb et al., Impact of common comorbidities on antimicrobial consumption and mortality amongst critically ill COVID-19 patients: A retrospective two center study in Saudi Arabia, Clinical Infection in Practice, doi:10.1016/j.clinpr.2023.100229.
Yilgwan et al., Clinical profile and Predictors of Outcomes of Hospitalized Patients with Laboratory-Confirmed Severe Acute Respiratory Syndrome Coronavirus 2 in Nigeria: A Retrospective Analysis of 13 High Burden States in Nigeria, Nigerian Medical Journal, 64:2, nigerianmedjournal.org/index.php/nmj/article/view/174.
de Gonzalo-Calvo et al., A blood microRNA classifier for the prediction of ICU mortality in COVID-19 patients: a multicenter validation study, Respiratory Research, doi:10.1186/s12931-023-02462-x.
Cárdenas-Jaén et al., Gastrointestinal symptoms and complications in patients hospitalized due to COVID-19, an international multicentre prospective cohort study (TIVURON project), Gastroenterología y Hepatología (English Edition), doi:10.1016/j.gastre.2023.05.002.
Shamsi et al., Survival and Mortality in Hospitalized Children with COVID-19: A Referral Center Experience in Yazd, Iran, Canadian Journal of Infectious Diseases and Medical Microbiology, doi:10.1155/2023/5205188.
Afşin et al., Factors affecting prognosis and mortality in severe COVID-19 pneumonia patients, Acta Clinica Croatica, doi:10.20471/acc.2023.62.01.13.
Burhan et al., Characteristics and outcomes of patients with severe COVID-19 in Indonesia: Lessons from the first wave, PLOS ONE, doi:10.1371/journal.pone.0290964.
Meeus et al., Efficacy and safety of in-hospital treatment of Covid-19 infection with low-dose hydroxychloroquine and azithromycin in hospitalized patients: A retrospective controlled cohort study, New Microbes and New Infections, doi:10.1016/j.nmni.2023.101172.
Souza-Silva et al., Dados de Vida Real sobre o Uso da Hidroxicloroquina ou da Cloroquina Combinadas ou Não à Azitromicina em Pacientes com Covid-19: Uma Análise Retrospectiva no Brasil, Arquivos Brasileiros de Cardiologia, doi:10.36660/abc.20220935.
Mehrizi et al., Drug prescription patterns and their association with mortality and hospitalization duration in COVID-19 patients: insights from big data, Frontiers in Public Health, doi:10.3389/fpubh.2023.1280434.
AlShehhi et al., Utilizing machine learning for survival analysis to identify risk factors for COVID-19 intensive care unit admission: A retrospective cohort study from the United Arab Emirates, PLOS ONE, doi:10.1371/journal.pone.0291373.
Değirmenci et al., Is vitamin D level important in pregnant women with COVID-19?, Journal of Controversies in Obstetrics & Gynecology and Pediatrics, doi:10.51271/JCOGP-0035.
Brouqui et al., SARS-CoV 2 Viral Clearance in 1276 Patients: Associated Factors and the Role of Treatment with Hydroxychloroquine and Azithromycin, Acta Scientific Microbiology, doi:10.31080/ASMI.2024.07.1413.
Azimi Pirsaraei et al., Anticoagulant Use in COVID-19 Patients: A Longitudinal Study From Zanjan, Iran, Cureus, doi:10.7759/cureus.66798.
Kim (B) et al., One-year mortality and associated factors in older hospitalized COVID-19 survivors: a Nationwide Cohort Study in Korea, Scientific Reports, doi:10.1038/s41598-024-76871-3.
He et al., Clinical characteristics and risk factors for in-hospital mortality of COVID-19 patients in Hubei Province: A multicenter retrospective study, IJC Heart & Vasculature, doi:10.1016/j.ijcha.2024.101574.
Dinoi et al., Retrospective Clinical Investigation into the Association Between Abnormal Blood Clotting, Oral Anticoagulant Therapy, and Medium-Term Mortality in a Cohort of COVID-19 Patients, Biomedicines, doi:10.3390/biomedicines13030535.
He (B) et al., Low dose of hydroxychloroquine is associated with reduced COVID-19 mortality: a multicenter study in China, Frontiers of Medicine, doi:10.1007/s11684-025-1123-9.
Alqahtani et al., Outcomes of COVID-19 During the First Wave in Saudi Arabia: An Observational Study of ICU Cases from a Single Hospital, Journal of Clinical Medicine, doi:10.3390/jcm14061915.
Gendelman et al., Continuous Hydroxychloroquine or Colchicine Therapy Does Not Prevent Infection With SARS-CoV-2: Insights From a Large Healthcare Database Analysis, Autoimmunity Reviews, 19:7, July 2020, doi:10.1016/j.autrev.2020.102566.
Konig et al., Baseline use of hydroxychloroquine in systemic lupus erythematosus does not preclude SARS-CoV-2 infection and severe COVID-19, Annals of the Rheumatic Diseases, doi:10.1136/annrheumdis-2020-217690.
Cassione et al., COVID-19 infection in a northern-Italian cohort of systemic lupus erythematosus assessed by telemedicine, Annals of the Rheumatic Diseases, doi:10.1136/annrheumdis-2020-217717.
Macias et al., Similar incidence of Coronavirus Disease 2019 (COVID-19) in patients with rheumatic diseases with and without hydroxychloroquine therapy, medRxiv, doi:10.1101/2020.05.16.20104141.
Gianfrancesco et al., Characteristics associated with hospitalisation for COVID-19 in people with rheumatic disease: data from the COVID-19 Global Rheumatology Alliance physician-reported registry, Annals of the Rheumatic Diseases, 79:7, 859-866, doi:10.1136/annrheumdis-2020-217871.
Chatterjee et al., Healthcare workers & SARS-CoV-2 infection in India: A case-control investigation in the time of COVID-19, Indian Journal of Medical Research, doi:10.4103/ijmr.IJMR_2234_20.
Bhattacharya et al., Pre exposure Hydroxychloroquine use is associated with reduced COVID19 risk in healthcare workers, medRxiv, doi:10.1101/2020.06.09.20116806.
Huang (C) et al., Clinical characteristics of 17 patients with COVID-19 and systemic autoimmune diseases: a retrospective study, Annals of the Rheumatic Diseases, doi:10.1136/annrheumdis-2020-217425.
Gendebien et al., Systematic analysis of COVID-19 infection and symptoms in a systemic lupus erythematosus population: correlation with disease characteristics, hydroxychloroquine use and immunosuppressive treatments, Annals of the Rheumatic Diseases, doi:10.1136/annrheumdis-2020-218244.
Ferreira (C) et al., Chronic treatment with hydroxychloroquine and SARS-CoV-2 infection, Journal of Medical Virology, doi:10.1002/jmv.26286.
Zhong et al., COVID-19 in patients with rheumatic disease in Hubei province, China: a multicentre retrospective observational study, Lancent Rheumatology, doi:10.1016/S2665-9913(20)30227-7.
Desbois et al., Prevalence and clinical features of COVID-19 in a large cohort of 199 patients with sarcoidosis, Research Square, doi:10.21203/rs.3.rs-41653/v1.
Kadnur et al., Hydroxychloroquine pre-exposure prophylaxis for COVID-19 among healthcare workers: Initial experience from India, Journal of Family Medicine and Primary Care, doi:10.4103/jfmpc.jfmpc_1177_21.
Khurana et al., Prevalence and clinical correlates of COVID-19 outbreak among healthcare workers in a tertiary level hospital, medRxiv, doi:10.1101/2020.07.21.20159301.
Santos (B) et al., Determinants of COVID-19 disease severity in patients with underlying rheumatic disease, Clinical Rheumatology, doi:10.1007/s10067-020-05301-2.
Singer et al., Hydroxychloroquine ineffective for COVID-19 prophylaxis in lupus and rheumatoid arthritis, Annals of the Rheumatic Diseases, doi:10.1136/annrheumdis-2020-218500.
Salvarani et al., Susceptibility to COVID-19 in Patients Treated With Antimalarials: A Population-Based Study in Emilia-Romagna, Northern Italy, Arthritis & Rheumatology, doi:10.1002/art.41475.
Piñana et al., Risk factors and outcome of COVID-19 in patients with hematological malignancies, Experimental Hematology & Oncology, doi:10.1186/s40164-020-00177-z.
Ferri et al., COVID-19 and rheumatic autoimmune systemic diseases: report of a large Italian patients series, Clinical Rheumatology, doi:10.1007/s10067-020-05334-7.
de la Iglesia et al., Hydroxicloroquine for pre-exposure prophyylaxis for SARS-CoV-2, medRxiv, doi:10.1101/2020.08.31.20185314.
Laplana et al., Lack of protective effect of chloroquine derivatives on COVID-19 disease in a Spanish sample of chronically treated patients, PLOS ONE, doi:10.1371/journal.pone.0243598.
Rentsch et al., Effect of pre-exposure use of hydroxychloroquine on COVID-19 mortality: a population-based cohort study in patients with rheumatoid arthritis or systemic lupus erythematosus using the OpenSAFELY platform, The Lancet Rheumatology, doi:10.1016/S2665-9913(20)30378-7.
Grau-Pujol et al., Pre-exposure prophylaxis with hydroxychloroquine for COVID-19: a double-blind, placebo-controlled randomized clinical trial, Trials, doi:10.1186/s13063-021-05758-9.
Rajasingham et al., Hydroxychloroquine as pre-exposure prophylaxis for COVID-19 in healthcare workers: a randomized trial, Clinical Infectious Diseases, doi:10.1093/cid/ciaa1571.
Gentry et al., Long-term hydroxychloroquine use in patients with rheumatic conditions and development of SARS-CoV-2 infection: a retrospective cohort study, Lancet Rheumatology, doi:10.1016/S2665-9913(20)30305-2.
Abella et al., Efficacy and Safety of Hydroxychloroquine vs Placebo for Pre-exposure SARS-CoV-2 Prophylaxis Among Health Care Workers, JAMA Internal Medicine, doi:10.1001/jamainternmed.2020.6319.
Yadav et al., Sero-survey for health-care workers provides corroborative evidence for the effectiveness of Hydroxychloroquine prophylaxis against COVID-19 infection, ResearchGate, doi:10.13140/RG.2.2.34411.77603.
Goenka et al., Seroprevalence of COVID-19 Amongst Health Care Workers in a Tertiary Care Hospital of a Metropolitan City from India, SSRN, doi:10.2139/ssrn.3689618.
Arleo et al., Clinical Course and Outcomes of coronavirus disease 2019 (COVID-19) in Rheumatic Disease Patients on Immunosuppression: A case Cohort Study at a Single Center with a Significantly Diverse Population, medRxiv, doi:10.1101/2020.10.26.20219154.
Behera (C) et al., Role of ivermectin in the prevention of SARS-CoV-2 infection among healthcare workers in India: A matched case-control study, PLOS ONE, doi:10.1371/journal.pone.0247163.
Datta et al., No Role of HCQ in COVID-19 Prophylaxis: A Survey amongst Indian Doctors, Journal of Vaccines & Vaccination, S6:1000002, www.longdom.org/open-access/no-role-of-hcq-in-covid19-prophylaxis-a-survey-amongst-indian-doctors.pdf.
Mathai et al., Hydroxychloroquine as pre-exposure prophylaxis against COVID-19 in health-care workers: A single-center experience, Journal of Marine Medical Society, doi:10.4103/jmms.jmms_115_20.
Revollo et al., Hydroxychloroquine pre-exposure prophylaxis for COVID-19 in healthcare workers, Journal of Antimicrobial Chemotherapy, doi:10.1093/jac/dkaa477.
Jung et al., Effect of hydroxychloroquine pre-exposure on infection with SARS-CoV-2 in rheumatic disease patients: A population-based cohort study, Clinical Microbiology and Infection, doi:10.1016/j.cmi.2020.12.003.
Gönenli et al., Analysis of the Prophylactic use of Hydroxychloroquine at the Beginning of the COVID-19 Pandemic Among Physicians, Infectious Diseases and Clinical Microbiology, doi:10.36519/idcm.2022.111.
Huh et al., Association of prescribed medications with the risk of COVID-19 infection and severity among adults in South Korea, International Journal of Infectious Diseases, doi:10.1016/j.ijid.2020.12.041.
Cordtz et al., Incidence and severeness of COVID-19 hospitalisation in patients with inflammatory rheumatic disease: a nationwide cohort study from Denmark, Rheumatology, doi:10.1093/rheumatology/keaa897.
Rangel et al., Chronic Hydroxychloroquine Therapy and COVID-19 Outcomes: A Retrospective Case-Control Analysis, Journal of the American Academy of Dermatology, doi:10.1016/j.jaad.2020.10.098.
Khoubnasabjafari et al., Prevalence of COVID-19 in patients with rheumatoid arthritis (RA) already treated with hydroxychloroquine (HCQ) compared with HCQ-naive patients with RA: a multicentre cross-sectional study, Postgraduate Medical Journal, doi:10.1136/postgradmedj-2020-139561.
Trefond et al., Effet d’un traitement par hydroxychloroquine prescrit comme traitement de fond de rhumatismes inflammatoires chroniques ou maladies auto-immunes systémiques sur les tests diagnostiques et l’évolution de l’infection à SARS CoV-2: étude de 871 patients, Revue du Rhumatisme, doi:10.1016/j.rhum.2021.09.004.
Strangfeld et al., Factors associated with COVID-19-related death in people with rheumatic diseases: results from the COVID-19 Global Rheumatology Alliance physician-reported registry, Annals of the Rheumatic Diseases, doi:10.1136/annrheumdis-2020-219498.
Fitzgerald et al., Risk Factors for Infection and Health Impacts of the COVID-19 Pandemic in People with Autoimmune Diseases, medRxiv, doi:10.1101/2021.02.03.21251069.
Mahto et al., Seroprevalence of IgG against SARS-CoV-2 and its determinants among healthcare workers of a COVID-19 dedicated hospital of India, American Journal of Blood Research, 11:1, www.ncbi.nlm.nih.gov/labs/pmc/articles/PMC8010601/.
Bae et al., Recent Hydroxychloroquine Use Is Not Significantly Associated with Positive PCR Results for SARS-CoV-2: A Nationwide Observational Study in South Korea, Viruses 2021, doi:10.3390/v13020329.
Pham et al., Failure of chronic hydroxychloroquine in preventing severe complications of COVID-19 in patients with rheumatic diseases, Rheumatology Advances in Practice, doi:10.1093/rap/rkab014.
Vivanco-Hidalgo et al., Incidence of COVID-19 in patients exposed to chloroquine and hydroxychloroquine: results from a population-based prospective cohort in Catalonia, Spain, 2020, Eurosurveillance, doi:10.2807/1560-7917.ES.2021.26.9.2001202.
Dev et al., Risk factors and frequency of COVID-19 among healthcare workers at a tertiary care centre in India: a case–control study, Transactions of The Royal Society of Tropical Medicine and Hygiene, doi:10.1093/trstmh/trab047.
Seet (B) et al., Positive impact of oral hydroxychloroquine and povidone-iodine throat spray for COVID-19 prophylaxis: an open-label randomized trial, International Journal of Infectious Diseases, doi:10.1016/j.ijid.2021.04.035.
Alegiani et al., Risk of COVID-19 hospitalization and mortality in rheumatic patients treated with hydroxychloroquine or other conventional DMARDs in Italy, Rheumatology, doi:10.1093/rheumatology/keab348.
Alzahrani et al., Clinical characteristics and outcome of COVID-19 in patients with rheumatic diseases, Rheumatology International , doi:10.1007/s00296-021-04857-9.
Rojas-Serrano et al., Hydroxychloroquine for prophylaxis of COVID-19 in health workers: A randomized clinical trial, PLOS ONE, doi:10.1371/journal.pone.0261980.
Syed et al., Pre-exposure Prophylaxis With Various Doses of Hydroxychloroquine Among Healthcare Personnel With High-Risk Exposure to COVID-19: A Randomized Controlled Trial, Cureus, doi:10.7759/cureus.20572.
Kamstrup et al., Hydroxychloroquine as a primary prophylactic agent against sars-cov-2 infection: a cohort study, International Journal of Infectious Diseases, doi:10.1016/j.ijid.2021.05.076.
Korkmaz et al., The effect of Hydroxychloroquine use due to rheumatic disease on the risk of Covid-19 infection and its course, Authorea, doi:10.22541/au.162257516.68665404/v1.
Badyal et al., Hydroxychloroquine for SARS CoV2 Prophylaxis in Healthcare Workers – A Multicentric Cohort Study Assessing Effectiveness and Safety, Journal of the Association of Physicians of India, 69:6, June 2021, www.researchgate.net/publication/357700064_Hydroxychloroquine_for_SARS_CoV2_Prophylaxis_in_Healthcare_Workers_-_A_Multicentric_Cohort_Study_Assessing_Effectiveness_and_Safety.
Shaw et al., COVID-19 in Individuals Treated With Long-Term Hydroxychloroquine: A Propensity Score-Matched Analysis of Cicatricial Alopecia Patients, Journal of Drugs in Dermatology, doi:10.36849/JDD.5843.
Küçükakkaş et al., The effect of hydroxychloroquine against SARS-CoV-2 infection in rheumatoid arthritis patients, Research Square, doi:10.21203/rs.3.rs-43812/v1.
Bhatt et al., Hydroxychloroquine Prophylaxis against Coronavirus Disease-19: Practice Outcomes among Health-Care Workers, medRxiv, doi:10.1101/2021.08.02.21260750.
McCullough et al., Hydroxychloroquine in the Prevention of COVID-19 Infection in Healthcare Workers, NCT04333225, clinicaltrials.gov/study/NCT04333225.
Patil et al., A Prospective Longitudinal Study Evaluating The Influence of Immunosuppressives and Other Factors On COVID-19 in Autoimmune Rheumatic Diseases, Research Square, doi:10.21203/rs.3.rs-805748/v1.
Naggie (B) et al., Hydroxychloroquine for pre-exposure prophylaxis of COVID-19 in health care workers: A randomized, multicenter, placebo-controlled trial (HERO-HCQ), International Journal of Infectious Diseases, doi:10.1016/j.ijid.2023.01.019.
Cordtz (B) et al., Incidence of COVID-19 Hospitalisation in Patients with Systemic Lupus Erythematosus: A Nationwide Cohort Study from Denmark, Journal of Clinical Medicine, doi:10.3390/jcm10173842.
Agarwal et al., Low dose hydroxychloroquine prophylaxis for COVID-19 - a prospective study, medRxiv, doi:10.1101/2021.09.13.21262971.
Guillaume et al., Antirheumatic Drug Intake Influence on Occurrence of COVID-19 Infection in Ambulatory Patients with Immune-Mediated Inflammatory Diseases: A Cohort Study, Rheumatology and Therapy, doi:10.1007/s40744-021-00373-1.
Fung et al., Effect of common maintenance drugs on the risk and severity of COVID-19 in elderly patients, PLoS ONE, doi:10.1371/journal.pone.0266922.
Belmont et al., COVID-19 PrEP HCW HCQ Study, ClinicalTrials.gov, NCT04354870, clinicaltrials.gov/ct2/show/results/NCT04354870.
Samajdar (B) et al., Ivermectin and Hydroxychloroquine for Chemo-Prophylaxis of COVID-19: A Questionnaire Survey of Perception and Prescribing Practice of Physicians vis-a-vis Outcomes, Journal of the Association of Physicians India, 69:11, www.researchgate.net/publication/356294136_Ivermectin_and_Hydroxychloroquine_for_Chemo-Prophylaxis_of_COVID-19_A_Questionnaire_Survey_of_Perception_and_Prescribing_Practice_of_Physicians_vis-a-vis_Outcomes.
Ahmed (B) et al., Factors Affecting the Incidence, Progression, and Severity of COVID-19 in Type 1 Diabetes Mellitus, BioMed Research International, doi:10.1155/2021/1676914.
Rao et al., Hydroxychloroquine as pre-exposure prophylaxis against COVID-19 infection among healthcare workers: a prospective cohort study, Expert Review of Anti-infective Therapy, doi:10.1080/14787210.2022.2015326.
McKinnon et al., Safety and Tolerability of Hydroxychloroquine in healthcare workers and first responders for the prevention of COVID-19: WHIP COVID-19 Study, International Journal of Infectious Diseases, doi:10.1016/j.ijid.2021.12.343.
Juneja et al., Hydroxychloroquine pre-exposure prophylaxis provides no protection against COVID-19 among health care workers: a cross-sectional study in a tertiary care hospital in North India, Journal of Basic and Clinical Physiology and Pharmacology, doi:10.1515/jbcpp-2021-0221.
Erden et al., COVID-19 outcomes in patients with antiphospholipid syndrome: a retrospective cohort study, Bratislava Medical Journal, doi:10.4149/BLL_2022_018.
Ugarte-Gil et al., Characteristics associated with poor COVID-19 outcomes in individuals with systemic lupus erythematosus: data from the COVID-19 Global Rheumatology Alliance, Annals of the Rheumatic Diseases, doi:10.1136/annrheumdis-2021-221636.
Opdam et al., Identification of Risk Factors for COVID-19 Hospitalization in Patients with Anti-Rheumatic Drugs: Results from a Multicenter Nested Case Control Study, Clinical Pharmacology & Therapeutics, doi:10.1002/cpt.2551.
Oztas et al., Frequency and Severity of COVID-19 in Patients with Various Rheumatic Diseases Treated Regularly with Colchicine or Hydroxychloroquine, Journal of Medical Virology, doi:10.1002/jmv.27731.
MacFadden et al., Screening Large Population Health Databases for Potential COVID-19 Therapeutics: A Pharmacopeia-Wide Association Study (PWAS) of Commonly Prescribed Medications, Open Forum Infectious Diseases, doi:10.1093/ofid/ofac156.
Satti et al., Characteristics and Obstetric Outcomes in Women With Autoimmune Rheumatic Disease During the COVID-19 Pandemic in Qatar, Cureus, doi:10.7759/cureus.24382.
Tirupakuzhi Vijayaraghavan et al., Hydroxychloroquine plus personal protective equipment versus personal protective equipment alone for the prevention of laboratory-confirmed COVID-19 infections among healthcare workers: a multicentre, parallel-group randomised controlled trial from India, BMJ Open, doi:10.1136/bmjopen-2021-059540.
Raabe et al., Hydroxychloroquine pre-exposure prophylaxis to prevent SARS-CoV-2 among health care workers at risk for SARS-CoV-2 exposure: A nonrandomized controlled trial, medRxiv, doi:10.1101/2022.07.01.22277058.
Yadav (B) et al., Hydroxychloroquine/chloroquine prophylaxis among health-care workers: Was it really preventive? – Evidence from a multicentric cross-sectional study, Indian Journal of Community Medicine, doi:10.4103/ijcm.ijcm_684_21.
Patel et al., Factors Associated with COVID-19 Breakthrough Infection in the Pre-Omicron Era Among Vaccinated Patients with Rheumatic Diseases: A Cohort Study, medRxiv, doi:10.1101/2022.07.13.22277606.
Polo et al., Daily tenofovir disoproxil fumarate/emtricitabine and hydroxychloroquine for pre-exposure prophylaxis of COVID-19: a double-blind placebo controlled randomized trial in healthcare workers, Clinical Microbiology and Infection, doi:10.1016/j.cmi.2022.07.006.
Becetti et al., Prevalence of coronavirus disease 2019 in a multiethnic cohort of patients with autoimmune rheumatic diseases in Qatar, Qatar Medical Journal, doi:10.5339/qmj.2022.37.
Loucera et al., Real-world evidence with a retrospective cohort of 15,968 COVID-19 hospitalized patients suggests 21 new effective treatments, Virology Journal, doi:10.1186/s12985-023-02195-9.
Oku et al., Risk factors for hospitalization or mortality for COVID-19 in patients with rheumatic diseases: Results of a nation-wide JCR COVID-19 registry in Japan, Modern Rheumatology, doi:10.1093/mr/roac104.
Sahebari et al., Influence of biologic and conventional disease-modifying antirheumatic drugs on COVID-19 incidence among rheumatic patients during the first and second wave of the pandemic in Iran, Reumatologia/Rheumatology, doi:10.5114/reum.2022.119039.
Obrișcă et al., Characteristics of SARS-CoV-2 Infection in an Actively Monitored Cohort of Patients with Lupus Nephritis, Biomedicines, doi:10.3390/biomedicines10102423.
Isnardi et al., Sociodemographic and clinical factors associated with poor COVID-19 outcomes in patients with rheumatic diseases: data from the SAR-COVID Registry, Clinical Rheumatology, doi:10.1007/s10067-022-06393-8.
Sukumar et al., The Frontline War: A Case-control study of risk factors for COVID-19 among health care workers, F1000Research, doi:10.12688/f1000research.109023.1.
Shahrin et al., Hospital-Based Quasi-Experimental Study on Hydroxychloroquine Pre-Exposure Prophylaxis for COVID-19 in Healthcare Providers with Its Potential Side-Effects, Life, doi:10.3390/life12122047.
Shukla et al., An observational multi-centric COVID-19 sequelae study among health care workers, The Lancet Regional Health - Southeast Asia, doi:10.1016/j.lansea.2022.100129.
Nasri et al., Efficacy of hydroxychloroquine in pre-exposure severe acute respiratory syndrome coronavirus 2 prophylaxis among high-risk healthcare workers: A multicenter study, Advanced Biomedical Research, doi:10.4103/abr.abr_104_21.
Llanos-Cuentas et al., Hydroxychloroquine to prevent SARS-CoV-2 infection among healthcare workers: early termination of a phase 3, randomised, open-label, controlled clinical trial, BMC Research Notes, doi:10.1186/s13104-023-06281-7.
Mathew et al., Predictors of COVID-19 severity and outcomes in Indian patients with rheumatic diseases: a prospective cohort study, Rheumatology Advances in Practice, doi:10.1093/rap/rkad025.
Chevalier et al., CovAID: Identification of factors associated with severe COVID-19 in patients with inflammatory rheumatism or autoimmune diseases, Frontiers in Medicine, doi:10.3389/fmed.2023.1152587.
Sen et al., Post-COVID-19 condition in patients with autoimmune rheumatic diseases: the COVID-19 Vaccination in Autoimmune Diseases (COVAD) study, The Lancet Rheumatology, doi:10.1016/S2665-9913(23)00066-8.
Dulcey et al., Long-Term Hydroxychloroquine and Its Association with Covid-19 Infection, a Cohort Study from a South American Hospital, Journal of Clinical Rheumatology, doi:10.1097/RHU.0000000000001986.
Alqatari et al., COVID-19 in patients with rheumatological diseases in the Eastern Province of Saudi Arabia, Journal of Medicine and Life, doi:10.25122/jml-2023-0037.
Finkelstein et al., The Efficacy of Long-Term Hydroxychloroquine Use in the Prevention of COVID-19: A Retrospective Cohort Study, Studies in Health Technology and Informatics, doi:10.3233/SHTI230489.
Klebanov et al., Antimalarials are not Effective as Pre-Exposure Prophylaxis for COVID-19: A Retrospective Matched Control Study, Journal of Drugs in Dermatology, doi:10.36849/jdd.6593.
Scirocco et al., COVID-19 prognosis in systemic lupus erythematosus compared with rheumatoid arthritis and spondyloarthritis: results from the CONTROL-19 Study by the Italian Society for Rheumatology, Lupus Science & Medicine, doi:10.1136/lupus-2023-000945.
Rabe et al., Impact of SARS-CoV-2 infection on patients with systemic lupus erythematosus in England prior to vaccination: a retrospective observational cohort study, BMJ Open, doi:10.1136/bmjopen-2022-071072.
Huang (D) et al., Effect of traditional therapeutics on prevalence and clinical outcomes of coronavirus disease 2019 in Chinese patients with autoimmune diseases, Journal of Translational Autoimmunity, doi:10.1016/j.jtauto.2023.100227.
Salesi et al., Clinical signs, symptoms, and severity of COVID-19 in patients with rheumatic diseases during the COVID-19 epidemic, Immunopathologia Persa, doi:10.34172/ipp.2023.40568.
Chouhdari et al., The prophylactic effect of hydroxychloroquine on the severity of COVID-19 infection in an asymptomatic population: A randomized clinical trial, Social Determinants of Health, doi:10.22037/sdh.v10i1.43032.
Liu et al., Factors affecting different COVID-19 outcomes in patients with systemic lupus erythematosus during the second pandemic wave of COVID-19 in China, Lupus, doi:10.1177/09612033241230736.
Dey et al., Hydroxy Chloroquine Prophylaxis Experience in Doctor Community with COVID-19 in West Bengal, Journal of College of Medical Sciences-Nepal, doi:10.3126/jcmsn.v20i2.43302.
Rutskaya-Moroshan et al., Clinical Characteristics, Prognostic Factors, and Outcomes of COVID-19 in Autoimmune Rheumatic Disease Patients: A Retrospective Case–Control Study from Astana, Kazakhstan, Medicina, doi:10.3390/medicina60091377.
Schilling (B) et al., Evaluation of hydroxychloroquine or chloroquine for the prevention of COVID-19 (COPCOV): A double-blind, randomised, placebo-controlled trial, PLOS Medicine, doi:10.1371/journal.pmed.1004428.
Patel (B) et al., Patients with systemic autoimmune rheumatic diseases remain at risk for hospitalisation for COVID-19 infection in the Omicron era (2022–2024): a retrospective cohort study, RMD Open, doi:10.1136/rmdopen-2024-005114.
Boulware et al., A Randomized Trial of Hydroxychloroquine as Postexposure Prophylaxis for Covid-19, NEJM, June 3 2020, doi:10.1056/NEJMoa2016638.
Mitjà (B) et al., A Cluster-Randomized Trial of Hydroxychloroquine as Prevention of Covid-19 Transmission and Disease, NEJM, doi:10.1056/NEJMoa2021801.
Polat et al., Hydroxychloroquine Use on Healthcare Workers Exposed to COVID-19 - A Pandemic Hospital Experience, Medical Journal of Bakirkoy, 16:3, 280-6, doi:10.5222/BMJ.2020.50469.
Dhibar et al., Post Exposure Prophylaxis with Hydroxychloroquine (HCQ) for the Prevention of COVID-19, a Myth or a Reality? The PEP-CQ Study, International Journal of Antimicrobial Agents, doi:10.1016/j.ijantimicag.2020.106224.
Simova (B) et al., Hydroxychloroquine for prophylaxis and treatment of COVID-19 in health care workers, New Microbes and New Infections, doi:10.1016/j.nmni.2020.100813.
Barnabas et al., Hydroxychloroquine for Post-exposure Prophylaxis to Prevent Acute Respiratory Syndrome Coronavirus 2 (SARS-CoV-2) Infection: A Randomized Trial, Annals of Internal Medicine, doi:10.7326/M20-6519.
Shabani et al., Evaluation of the Prophylactic Effect of Hydroxychloroquine on People in Close-Contact with Patients with Covid-19, Pulmonary Pharmacology & Therapeutics, doi:10.1016/j.pupt.2021.102069.
Dhibar (B) et al., The ‘myth of Hydroxychloroquine (HCQ) as post-exposure prophylaxis (PEP) for the prevention of COVID-19’ is far from reality, Scientific Reports, doi:10.1038/s41598-022-26053-w.
Reis (C) et al., Effect of early treatment with metformin on risk of emergency care and hospitalization among patients with COVID-19: The TOGETHER randomized platform clinical trial, The Lancet Regional Health - Americas, doi:10.1016/j.lana.2021.100142.
Bramante (B) et al., Randomized Trial of Metformin, Ivermectin, and Fluvoxamine for Covid-19, NEJM, doi:10.1056/NEJMoa2201662.
Bramante (C) et al., Metformin reduces the risk of Long COVID or Death over 6 months in an Emulated Target Trial of Primarily Omicron-infected Adults without Diabetes or Prediabetes: a New-User, Active-Comparator Analysis Using the National COVID Cohort Collaborative (N3C) Electronic Health Record Database. This research was supported in part by the Intramural/Extramural research program of the National Center for Advancing Translational Science, NIH, Open Forum Infectious Diseases, doi:10.1093/ofid/ofae631.016.
Abu-Jamous et al., Associations of comorbidities and medications with COVID-19 outcome: A retrospective analysis of real-world evidence data, medRxiv, doi:10.1101/2020.08.20.20174169.
Tamura (B) et al., Outcome and death risk of diabetes patients with Covid-19 receiving pre-hospital and in-hospital metformin therapies, Diabetology & Metabolic Syndrome, doi:10.1186/s13098-021-00695-8.
Li (C) et al., Inpatient use of metformin and acarbose is associated with reduced mortality of COVID-19 patients with type 2 diabetes mellitus, Endocrinology, Diabetes & Metabolism, doi:10.1002/edm2.301.
Shaseb et al., Long and Short-term Metformin Consumption as a Potential Therapy to Prevent Complications of COVID-19, Advanced Pharmaceutical Bulletin, doi:10.34172/apb.2023.066.
Ventura-López et al., Treatment with metformin glycinate reduces SARS-CoV-2 viral load: An in vitro model and randomized, double-blind, Phase IIb clinical trial, Biomedicine & Pharmacotherapy, doi:10.1016/j.biopha.2022.113223.
Sugimoto et al., Biguanides Associate with Decreased Early Mortality and Risk of Acute Kidney Injury In Hospitalized COVID-19 Patients: a nationwide retrospective cohort study in Japan, medRxiv, doi:10.1101/2024.07.20.24310736.
Bramante (D) et al., Metformin on Time to Sustained Recovery in Adults with COVID-19: The ACTIV-6 Randomized Clinical Trial, medRxiv, doi:10.1101/2025.01.13.25320485.
Luo (C) et al., Metformin Treatment Was Associated with Decreased Mortality in COVID-19 Patients with Diabetes in a Retrospective Analysis, The American Journal of Tropical Medicine and Hygiene, doi:10.4269/ajtmh.20-0375.
Cariou et al., Phenotypic characteristics and prognosis of inpatients with COVID-19 and diabetes: the CORONADO study, Diabetologia, doi:10.1007/s00125-020-05180-x.
Choi (B) et al., Clinical Characteristics and Disease Progression in Early-Stage COVID-19 Patients in South Korea, Journal of Clinical Medicine, doi:10.3390/jcm9061959.
Wang (B) et al., A tertiary center experience of multiple myeloma patients with COVID-19: lessons learned and the path forward, Journal of Hematology & Oncology, doi:10.1186/s13045-020-00934-x.
Chen (F) et al., Clinical Characteristics and Outcomes of Patients With Diabetes and COVID-19 in Association With Glucose-Lowering Medication, Diabetes Care, doi:10.2337/dc20-0660.
Kim (C) et al., The Clinical Characteristics and Outcomes of Patients with Moderate-to-Severe Coronavirus Disease 2019 Infection and Diabetes in Daegu, South Korea, Diabetes & Metabolism Journal, doi:10.4093/dmj.2020.0146.
Li (D) et al., Metformin Use in Diabetes Prior to Hospitalization: Effects on Mortality in Covid-19, Endocrine Practice, doi:10.4158/EP-2020-0466.
Mirani et al., Impact of Comorbidities and Glycemia at Admission and Dipeptidyl Peptidase 4 Inhibitors in Patients With Type 2 Diabetes With COVID-19: A Case Series From an Academic Hospital in Lombardy, Italy, Diabetes Care, doi:10.2337/dc20-1340.
Goodall et al., Risk factors for severe disease in patients admitted with COVID-19 to a hospital in London, England: a retrospective cohort study, Epidemiology and Infection, doi:10.1017/S0950268820002472.
Gao et al., Risk of Metformin in Patients With Type 2 Diabetes With COVID-19: A Preliminary Retrospective Report, Clinical and Translational Science, doi:10.1111/cts.12897.
Pérez-Belmonte et al., Mortality and other adverse outcomes in patients with type 2 diabetes mellitus admitted for COVID-19 in association with glucose-lowering drugs: a nationwide cohort study, BMC Medicine, doi:10.1186/s12916-020-01832-2.
Bramante (E) et al., Metformin and risk of mortality in patients hospitalised with COVID-19: a retrospective cohort analysis, The Lancet Healthy Longevity, doi:10.1016/S2666-7568(20)30033-7.
Sourij et al., COVID‐19 fatality prediction in people with diabetes and prediabetes using a simple score upon hospital admission, Diabetes, Obesity and Metabolism, doi:10.1111/dom.14256.
Lalau et al., Metformin use is associated with a reduced risk of mortality in patients with diabetes hospitalised for COVID-19, Diabetes & Metabolism, doi:10.1016/j.diabet.2020.101216.
Huh (B) et al., Association of prescribed medications with the risk of COVID-19 infection and severity among adults in South Korea, International Journal of Infectious Diseases, doi:10.1016/j.ijid.2020.12.041.
Ramos-Rincón et al., Association between prior cardiometabolic therapy and in-hospital mortality in very old patients with type 2 diabetes mellitus hospitalized due to COVID-19. A nationwide observational study in Spain, Research Square, doi:10.21203/rs.3.rs-133358/v1.
Crouse et al., Metformin Use Is Associated With Reduced Mortality in a Diverse Population With COVID-19 and Diabetes, Frontiers in Endocrinology, doi:10.3389/fendo.2020.600439.
Lally et al., Metformin is Associated with Decreased 30-Day Mortality Among Nursing Home Residents Infected with SARS-CoV2, Journal of the American Medical Directors Association, doi:10.1016/j.jamda.2020.10.031.
Oh et al., Metformin use and risk of COVID-19 among patients with type II diabetes mellitus: an NHIS-COVID-19 database cohort study, Acta Diabetologica, doi:10.1007/s00592-020-01666-7.
Wargny et al., Predictors of hospital discharge and mortality in patients with diabetes and COVID-19: updated results from the nationwide CORONADO study, Diabetologia, doi:10.1007/s00125-020-05351-w.
Bramante (F) et al., Outpatient metformin use is associated with reduced severity of COVID‐19 disease in adults with overweight or obesity, Journal of Medical Virology, doi:10.1002/jmv.26873.
Holt et al., Risk factors for developing COVID-19: a population-based longitudinal study (COVIDENCE UK), Thorax, doi:10.1136/thoraxjnl-2021-217487.
Khunti et al., Prescription of glucose-lowering therapies and risk of COVID-19 mortality in people with type 2 diabetes: a nationwide observational study in England, The Lancet Diabetes & Endocrinology, doi:10.1016/S2213-8587(21)00050-4.
Jiang et al., Association of metformin with mortality or ARDS in patients with COVID-19 and type 2 diabetes: A retrospective cohort study, Diabetes Research and Clinical Practice, doi:10.1016/j.diabres.2020.108619.
Ghany et al., Metformin is associated with lower hospitalizations, mortality and severe coronavirus infection among elderly medicare minority patients in 8 states in USA, Diabetes & Metabolic Syndrome: Clinical Research & Reviews, doi:10.1016/j.dsx.2021.02.022.
Alamgir et al., Drug repositioning candidates identified using in-silico quasi-quantum molecular simulation demonstrate reduced COVID-19 mortality in 1.5M patient records, medRxiv, doi:10.1101/2021.03.22.21254110.
Gálvez-Barrón et al., COVID-19: Clinical Presentation and Prognostic Factors of Severe Disease and Mortality in the Oldest-Old Population: A Cohort Study, Gerontology, doi:10.1159/000515159.
Ravindra et al., Retrospective Assessment of Treatments of Hospitalized Covid-19 Patients, medRxiv, doi:10.1101/2021.04.20.21255792.
Blanc et al., Therapeutic prevention of COVID-19 in elderly: a case–control study, GeroScience, doi:10.1007/s11357-021-00397-z.
Boye et al., Risk Factors Associated with COVID-19 Hospitalization and Mortality: A Large Claims-Based Analysis Among People with Type 2 Diabetes Mellitus in the United States, Diabetes Therapy, doi:10.1007/s13300-021-01110-1.
Cheng et al., Metformin Is Associated with Higher Incidence of Acidosis, but Not Mortality, in Individuals with COVID-19 and Pre-existing Type 2 Diabetes, Cell Metabolism, doi:10.1016/j.cmet.2020.08.013.
Wang (C) et al., Evaluation and management of COVID-19-related severity in people with type 2 diabetes, BMJ Open Diabetes Research & Care, doi:10.1136/bmjdrc-2021-002299.
Ando et al., Impact of overlapping risks of type 2 diabetes and obesity on coronavirus disease severity in the United States, Scientific Reports, doi:10.1038/s41598-021-96720-x.
Wander et al., Prior Glucose-Lowering Medication Use and 30-Day Outcomes Among 64,892 Veterans With Diabetes and COVID-19, Diabetes Care, doi:10.2337/dc21-1351.
Saygili et al., Preadmission usage of metformin and mortality in COVID-19 patients including the post-discharge period, Irish Journal of Medical Science, doi:10.1007/s11845-021-02823-9.
Ong et al., Association Between Metformin Use and Mortality Among Patients with Type 2 Diabetes Mellitus Hospitalized for COVID-19 Infection, Journal of the ASEAN Federation of Endocrine Societies, doi:10.15605/jafes.036.02.20.
Bliden et al., Metformin Use in Patients Hospitalized With COVID-19: Lower Inflammation, Oxidative Stress, and Thrombotic Risk Markers and Better Clinical Outcomes, Circulation, 144:A12228, www.ahajournals.org/doi/abs/10.1161/circ.144.suppl_1.12228.
Al-Salameh et al., The association between metformin treatment and COVID-19 outcomes according to metformin continuation during hospitalisation, Diabetes & Metabolism, doi:10.1016/j.diabet.2021.101297.
Wallace et al., Association of the patterns of use of medications with mortality of COVID-19 infection: a hospital-based observational study, BMJ Open, doi:10.1136/bmjopen-2021-050051.
Ojeda-Fernández et al., Metformin use is associated with a decrease in risk of hospitalization and mortality in COVID-19 diabetic patients: a population-based study in Lombardy, Diabetes, Obesity and Metabolism, doi:10.1111/dom.14648.
Fu et al., Prognostic Factors for COVID-19 Hospitalized Patients with Preexisting Type 2 Diabetes, International Journal of Endocrinology, doi:10.1155/2022/9322332.
Usman et al., Metformin use in patients hospitalized with COVID-19: lower inflammation, oxidative stress, and thrombotic risk markers and better clinical outcomes, Journal of Thrombosis and Thrombolysis, doi:10.1007/s11239-022-02631-7.
Wong et al., Glycemic Control and Clinical Outcomes in U.S. Patients With COVID-19: Data From the National COVID Cohort Collaborative (N3C) Database, Diabetes Care, doi:10.2337/dc21-2186.
Wong (B) et al., Metformin Use in Relation to Clinical Outcomes and Hyperinflammatory Syndrome Among COVID-19 Patients With Type 2 Diabetes: A Propensity Score Analysis of a Territory-Wide Cohort, Frontiers in Endocrinology, doi:10.3389/fendo.2022.810914.
MacFadden (B) et al., Screening Large Population Health Databases for Potential COVID-19 Therapeutics: A Pharmacopeia-Wide Association Study (PWAS) of Commonly Prescribed Medications, Open Forum Infectious Diseases, doi:10.1093/ofid/ofac156.
Ma et al., Metformin is associated with favorable outcomes in patients with COVID-19 and type 2 diabetes mellitus, Scientific Reports, doi:10.1038/s41598-022-09639-2.
Yeh et al., Hospitalization and mortality in patients with COVID-19 with or at risk of type 2 diabetes: data from five health systems in Pennsylvania and Maryland, BMJ Open Diabetes Research & Care, doi:10.1136/bmjdrc-2022-002774.
Hunt et al., Medications Associated with Lower Mortality in a SARS-CoV-2 Positive Cohort of 26,508 Veterans, Journal of General Internal Medicine, doi:10.1007/s11606-022-07701-3.
Cousins et al., Integrative analysis of functional genomic screening and clinical data identifies a protective role for spironolactone in severe COVID-19, Cell Reports Methods, doi:10.1016/j.crmeth.2023.100503.
Shestakova et al., Risk factors for COVID-19 case fatality rate in people with type 1 and type 2 diabetes mellitus: A nationwide retrospective cohort study of 235,248 patients in the Russian Federation, Frontiers in Endocrinology, doi:10.3389/fendo.2022.909874.
Loucera (B) et al., Real-world evidence with a retrospective cohort of 15,968 COVID-19 hospitalized patients suggests 21 new effective treatments, Virology Journal, doi:10.1186/s12985-023-02195-9.
Chan et al., Metformin is Associated with Reduced COVID-19 Severity in Patients with Prediabetes, medRxiv, doi:10.1101/2022.08.29.22279355.
Zaccardi et al., Ethnicity and risks of severe COVID-19 outcomes associated with glucose-lowering medications: A cohort study, Diabetes, Obesity and Metabolism, doi:10.1111/dom.14872.
Yip et al., Metformin Does Not Reduce Hospitalisation for COVID-19, SSRN Electronic Journal, doi:10.2139/ssrn.4225660.
Ouchi et al., Antidiabetic treatment and COVID-19 Outcomes: A population-based cohort study in primary health care in Catalonia during the first wave of the pandemic, Primary Care Diabetes, doi:10.1016/j.pcd.2022.10.001.
Morrison et al., COVID-19 outcomes in patients taking cardioprotective medications, PLOS ONE, doi:10.1371/journal.pone.0275787.
Mannucci et al., Infection Rates and Impact of Glucose Lowering Medications on the Clinical Course of COVID-19 in People with Type 2 Diabetes: A Retrospective Observational Study, Diabetes, Metabolic Syndrome and Obesity: Targets and Therapy, doi:10.2147/DMSO.S385646.
Milosavljevic et al., Evaluation of Glycemic Control and Predictors of Severe Illness and Death in Patients With Diabetes Hospitalized With COVID-19, Journal of Community Hospital Internal Medicine Perspectives, doi:10.55729/2000-9666.1127.
Miao et al., Metformin use and mortality and length of stay among hospitalized patients with type 2 diabetes and COVID-19: A multiracial, multiethnic, urban observational study, Frontiers in Endocrinology, doi:10.3389/fendo.2022.1002834.
Servais et al., Mortality-related risk factors of inpatients with diabetes and COVID-19: a multicenter retrospective study in Belgium, Annals of Endocrinology, doi:10.1016/j.ando.2023.08.002.
Pinchera et al., Diabetes and SARS-CoV-2 Infection: The Potential Role of Antidiabetic Therapy in the Evolution of COVID-19, Microorganisms, doi:10.3390/microorganisms11010145.
Sandhu et al., Outpatient medications associated with protection from COVID-19 hospitalization, PLOS ONE, doi:10.1371/journal.pone.0282961.
Yen et al., Metformin use before COVID-19 vaccination and the risks of COVID-19 incidence, medical utilization, and all-cause mortality in patients with type 2 diabetes mellitus, Diabetes Research and Clinical Practice, doi:10.1016/j.diabres.2023.110692.
Araldi et al., Effects of antidiabetic drugs on mortality risks in individuals with type 2 diabetes: A prospective cohort study of UK Biobank participants, medRxiv, doi:10.1101/2023.05.19.23290214.
Akinosoglou et al., COVID-19 Outcomes and Diabetes Mellitus: A Comprehensive Multicenter Prospective Cohort Study, Microorganisms, doi:10.3390/microorganisms11061416.
Alieva et al., Factors influencing the severity of COVID-19 course for patients with diabetes mellitus in tashkent: a retrospective cohort study, Obesity and metabolism, doi:10.14341/omet12801.
Obiri-Yeboah et al., Association of metformin, dipeptidyl dipeptidase-4 inhibitors, and insulin with COVID-19-related hospital outcomes in patients with type 2 diabetes, Endocrine Practice, doi:10.1016/j.eprac.2023.06.001.
Piarulli et al., Association of renin-angiotensin-aldosterone system inhibitors with best COVID-19 outcomes in a diabetic population of the Veneto Region (north-east Italy): a lesson for endemic phase?, Nutrition, Metabolism and Cardiovascular Diseases, doi:10.1016/j.numecd.2023.06.016.
Greco et al., The Impact of GLP-1 RAs and DPP-4is on Hospitalisation and Mortality in the COVID-19 Era: A Two-Year Observational Study, Biomedicines, doi:10.3390/biomedicines11082292.
Guo et al., Effects of Metformin on COVID-19 Patients with Type 2 Diabetes: A Retrospective Study, Diabetes, Metabolic Syndrome and Obesity, doi:10.2147/DMSO.S417925.
Zihono et al., Metformin Effectiveness in Reducing Mortality among Covid-19 Patients with Type 2 Diabetes Mellitus at a Tertiary Hospital in Indonesia, Folia Medica Indonesiana, doi:10.20473/fmi.v59i3.46944.
Farah et al., Prevalence and risk factors of COVID-19 infection, mortality, and post-infection lung fibrosis in patients with type 2 diabetes: a cross-sectional study, Journal of International Medical Research, doi:10.1177/03000605231198413.
Bidari et al., Development of a Scoring Method Based on a Chest CT Scan to Determine the Outcomes of COVID-19 Patients, Cureus, doi:10.7759/cureus.47354.
Miguel et al., Enhanced fatty acid oxidation through metformin and baicalin as therapy for COVID-19 and associated inflammatory states in lung and kidney, Redox Biology, doi:10.1016/j.redox.2023.102957.
Mamari et al., The effect of Chronic treatments of Type 2-diabetes mellitus on COVID-19 Morbidity and Symptoms Severity, Research Journal of Pharmacy and Technology, doi:10.52711/0974-360X.2023.00831.
Al-kuraishy et al., The potential therapeutic effect of metformin in type 2 diabetic patients with severe COVID-19, European Review for Medical and Pharmacological Sciences, doi:10.26355/eurrev_202312_34583.
Jang et al., Impact of Antidiabetic Drugs on Clinical Outcomes of COVID-19: A Nationwide Population-Based Study, Endocrinology and Metabolism, doi:10.3803/EnM.2024.1857.
Lewandowski et al., Insulin and Metformin Administration: Unravelling the Multifaceted Association with Mortality across Various Clinical Settings Considering Type 2 Diabetes Mellitus and COVID-19, Biomedicines, doi:10.3390/biomedicines12030605.
Silverii et al., Is Metformin Use Associated with a More Favorable COVID-19 Course in People with Diabetes?, Journal of Clinical Medicine, doi:10.3390/jcm13071874.
Dimnjaković et al., Association of anti-diabetic drugs and covid-19 outcomes in patients with diabetes mellitus type 2 and chronic kidney disease: Nationwide registry analysis, PLOS ONE, doi:10.1371/journal.pone.0301056.
Xu et al., Effects of different treatments for type 2 diabetes mellitus on mortality of coronavirus disease from 2019 to 2021 in China: a multi-institutional retrospective study, Molecular Biomedicine, doi:10.1186/s43556-024-00183-1.
Olawore et al., Risk of Post-Acute Sequelae of SARS-CoV-2 Infection (PASC) Among Patients with Type 2 Diabetes Mellitus on Anti-Hyperglycemic Medications, Clinical Epidemiology, doi:10.2147/CLEP.S458901.
Chen (G) et al., Metformin alleviates inflammatory response and severity rate of COVID-19 infection in elderly individuals, Translational Medicine of Aging, doi:10.1016/j.tma.2024.04.001.
Hussein et al., The Proportion and Associated Factors for Mortality among COVID-19 Infection with Diabetes in Iraq, The Review of Diabetic Studies, doi:10.1900/RDS.2024.20.12.
Johnson et al., Prevalent Metformin Use in Adults With Diabetes and the Incidence of Long COVID: An EHR-Based Cohort Study From the RECOVER Program, Diabetes Care, doi:10.2337/DCa24-0032.
Harmon et al., Preadmission Metformin Use Is Associated with Reduced Mortality in Patients with Diabetes Mellitus Hospitalized with COVID-19, Journal of General Internal Medicine, doi:10.1007/s11606-024-08864-x.
Sakamaki et al., Insights from a multicenter nationwide cohort analysis in Japan on the association of underlying conditions and pharmacological interventions with COVID-19 disease severity, Discover Public Health, doi:10.1186/s12982-024-00225-7.
Somasundaram et al., Metformin use and its association with various outcomes in COVID-19 patients with diabetes mellitus: a retrospective cohort study in a tertiary care facility, Annals of Medicine, doi:10.1080/07853890.2024.2425829.
Chertok Shacham et al., Impact of blood glucose control on clinical outcomes in type 2 diabetes patients hospitalized with COVID-19 infection, Diabetes and Vascular Disease Research, doi:10.1177/14791641241288390.
Matviichuk et al., Unveiling risk factors for post-COVID-19 syndrome development in people with type 2 diabetes, Frontiers in Endocrinology, doi:10.3389/fendo.2024.1459171.
Benfathallah et al., Does the Consumption of Metformin Correlate With a Reduction in Mortality Among Patients With Type 2 Diabetes and COVID-19 in Morocco?, Cureus, doi:10.7759/cureus.77288.
Soff et al., Association of glycemic control with Long COVID in patients with type 2 diabetes: findings from the National COVID Cohort Collaborative (N3C), BMJ Open Diabetes Research & Care, doi:10.1136/bmjdrc-2024-004536.
Cadegiani (C) et al., An open-label prospective observational study of antiandrogen and non-antiandrogen early pharmacological approaches in females with mild-to-moderate COVID-19. The Pre-AndroCoV Female Trial, medRxiv, doi:10.1101/2020.10.05.20206870.
McCoy et al., Proxalutamide (GT0918) Reduces the Rate of Hospitalization for COVID-19 Male Outpatients: A Randomized Double-Blinded Placebo-Controlled Trial, Frontiers in Medicine, doi:10.3389/fmed.2021.668698.
Cadegiani (D) et al., Early Antiandrogen Therapy With Dutasteride Reduces Viral Shedding, Inflammatory Responses, and Time-to-Remission in Males With COVID-19: A Randomized, Double-Blind, Placebo-Controlled Interventional Trial (EAT-DUTA AndroCoV Trial – Biochemical), Cureus, doi:10.7759/cureus.13047.
Cadegiani (E) et al., Proxalutamide (GT0918) Reduces the Rate of Hospitalization in mild-to-moderate COVID-19 Female Patients: A Randomized Double-Blinded Placebo-Controlled Two-Arm Parallel Trial, medRxiv, doi:10.1101/2021.07.06.21260086.
Kintor, Kintor Pharma's Proxalutamide Demonstrated Reduction in Hospitalization/Mortality for Patients with Mild to Moderate COVID-19 in Phase III MRCT Study, Press Release, www.prnewswire.com/news-releases/kintor-pharmas-proxalutamide-demonstrated-reduction-in-hospitalizationmortality-for-patients-with-mild-to-moderate-covid-19-in-phase-iii-mrct-study-301518525.html.
Hunt (B) et al., Medications Associated with Lower Mortality in a SARS-CoV-2 Positive Cohort of 26,508 Veterans, Journal of General Internal Medicine, doi:10.1007/s11606-022-07701-3.
Vicenzi et al., The Efficacy of the Mineralcorticoid Receptor Antagonist Canrenone in COVID-19 Patients, Journal of Clinical Medicine, doi:10.3390/jcm9092943.
Goren et al., Anti-androgens may protect against severe COVID-19 outcomes: results from a prospective cohort study of 77 hospitalized men, Journal of the European Academy of Dermatology and Venereology, doi:10.1111/jdv.16953.
Mareev et al., Results of Open-Label non-Randomized Comparative Clinical Trial: “BromhexIne and Spironolactone for CoronаvirUs Infection requiring hospiTalization (BISCUIT), Кардиология, doi:10.18087/cardio.2020.11.n1440.
Zarehoseinzade et al., Finasteride in hospitalized adult males with COVID-19: A risk factor for severity of the disease or an adjunct treatment: A randomized controlled clinical trial, Medical Journal of The Islamic Republic of Iran, doi:10.47176/mjiri.35.30.
Ghandehari et al., Progesterone in Addition to Standard of Care vs Standard of Care Alone in the Treatment of Men Hospitalized With Moderate to Severe COVID-19, Chest, doi:10.1016/j.chest.2021.02.024.
Ersoy et al., Assessment Of The Efficacy Of Spironolactone For COVID-19 ARDS Patients, Aydin Sağlik Dergi̇si̇, doi:10.17932/IAU.ASD.2015.007/asd_v07i3002.
Welén et al., A Phase 2 Trial of the Effect of Antiandrogen Therapy on COVID-19 Outcome: No Evidence of Benefit, Supported by Epidemiology and In Vitro Data, European Urology, doi:10.1016/j.eururo.2021.12.013.
Cadegiani (F) et al., Final Results of a Randomized, Placebo-Controlled, Two-Arm, Parallel Clinical Trial of Proxalutamide for Hospitalized COVID-19 Patients: A Multiregional, Joint Analysis of the Proxa-Rescue AndroCoV Trial, Cureus, doi:10.7759/cureus.20691.
Davarpanah et al., Combination of spironolactone and sitagliptin improves clinical outcomes of outpatients with COVID-19: a prospective cohort study, Journal of Endocrinological Investigation, doi:10.1007/s40618-023-02141-0.
Kotfis et al., Mineralocorticoid Receptor Antagonist (Potassium Canrenoate) Does Not Influence Outcome in the Treatment of COVID-19-Associated Pneumonia and Fibrosis—A Randomized Placebo Controlled Clinical Trial, Pharmaceuticals, doi:10.3390/ph15020200.
Abbasi et al., A Randomized Trial of Sitagliptin and Spironolactone With Combination Therapy in Hospitalized Adults With COVID-19, Journal of the Endocrine Society, doi:10.1210/jendso/bvac017.
Gomaa et al., Advancing combination treatment with glycyrrhizin and boswellic acids for hospitalized patients with moderate COVID-19 infection: a randomized clinical trial, Inflammopharmacology, doi:10.1007/s10787-022-00939-7.
Hsieh et al., Efficacy and Safety of Complementary Therapy With Jing Si Herbal Tea in Patients With Mild-To-Moderate COVID-19: A Prospective Cohort Study, Frontiers in Nutrition, doi:10.3389/fnut.2022.832321.
Nickols et al., Effect of Androgen Suppression on Clinical Outcomes in Hospitalized Men With COVID-19, JAMA Network Open, doi:10.1001/jamanetworkopen.2022.7852.
Gordon, M., Phase 2 study of oral sabizabulin for the treatment of SARS-CoV-2 in hospitalized patients at high risk for ARDS, European Congress of Clinical Microbiology & Infectious Diseases, verupharma.com/news/veru-announces-oral-late-breaking-presentation-of-phase-2-data-of-sabizabulin-for-the-treatment-of-hospitalized-severe-covid-19-patients-at-high-risk-for-acute-respiratory-distress-syndrome-at-the-32n/.
Nicastri et al., A phase 2 randomized, double-blinded, placebo-controlled, multicenter trial evaluating the efficacy and safety of raloxifene for patients with mild to moderate COVID-19, eClinicalMedicine, doi:10.1016/j.eclinm.2022.101450.
Wadhwa et al., Phase 2 randomised placebo-controlled trial of spironolactone and dexamethasone versus dexamethasone in COVID-19 hospitalised patients in Delhi, medRxiv, doi:10.1101/2022.07.01.22277163.
Barnette et al., Oral Sabizabulin for High-Risk, Hospitalized Adults with Covid-19: Interim Analysis, NEJM Evidence, doi:10.1056/EVIDoa2200145.
Montopoli et al., Androgen-deprivation therapies for prostate cancer and risk of infection by SARS-CoV-2: a population-based study (N = 4532), Annals of Oncology, doi:10.1016/j.annonc.2020.04.479.
Holt (B) et al., Influence of inhibitors of the renin–angiotensin system on risk of acute respiratory distress syndrome in Danish hospitalized COVID-19 patients, Journal of Hypertension, doi:10.1097/hjh.0000000000002515.
Koskinen et al., Androgen deprivation and SARS-CoV-2 in men with prostate cancer, Annals of Oncology, doi:10.1016/j.annonc.2020.06.015.
Patel (C) et al., Does androgen deprivation therapy protect against severe complications from COVID-19?, Annals of Oncology, doi:10.1016/j.annonc.2020.06.023.
Bennani et al., Androgen deprivation therapy may constitute a more effective COVID-19 prophylactic than therapeutic strategy, Annals of Oncology, doi:10.1016/j.annonc.2020.08.2095.
Ianhez et al., Androgen sensitivity in COVID‐19 and antiandrogens: Prospective data are still needed, Dermatologic Therapy, doi:10.1111/dth.14166.
Lazzeri et al., Impact of anti-androgen therapies on COVID-19 susceptibility: a case-control study in male population from two COVID-19 regional centers of Lombardy (Italy), medRxiv, doi:10.1101/2020.04.20.20068056.
Kwon et al., Androgen-deprivation therapy and SARS-CoV-2 in men with prostate cancer: findings from the University of California Health System registry, Annals of Oncology, doi:10.1016/j.annonc.2021.01.067.
Klein et al., Androgen Deprivation Therapy in Men with Prostate Cancer Does Not Affect Risk of Infection with SARS-CoV-2, Journal of Urology, doi:10.1097/JU.0000000000001338.
Jeon et al., Effect of Spironolactone on COVID-19 in Patients With Underlying Liver Cirrhosis: A Nationwide Case-Control Study in South Korea, Frontiers in Medicine, doi:10.3389/fmed.2021.629176.
Shaw (B) et al., COVID-19 in Individuals Treated With Long-Term Hydroxychloroquine: A Propensity Score-Matched Analysis of Cicatricial Alopecia Patients, Journal of Drugs in Dermatology, doi:10.36849/JDD.5843.
Israel et al., Identification of drugs associated with reduced severity of COVID-19: A case-control study in a large population, Epidemiology and Global Health Microbiology and Infectious Disease, doi:10.7554/eLife.68165.
Jiménez-Alcaide et al., Influence of androgen deprivation therapy on the severity of COVID-19 in prostate cancer patients, The Prostate, doi:10.1002/pros.24232.
Kazan et al., The clinical impact of androgen deprivation therapy on SARS-CoV-2 infection rates and disease severity, Türk Üroloji Dergisi/Turkish Journal of Urology, doi:10.5152/tud.2021.21278.
Schmidt (B) et al., Association Between Androgen Deprivation Therapy and Mortality Among Patients With Prostate Cancer and COVID-19, JAMA Network Open, doi:10.1001/jamanetworkopen.2021.34330.
Duarte et al., Impact of androgen deprivation therapy on mortality of prostate cancer patients with COVID-19: a propensity score-based analysis, Infectious Agents and Cancer, doi:10.1186/s13027-021-00406-y.
Welén (B) et al., A Phase 2 Trial of the Effect of Antiandrogen Therapy on COVID-19 Outcome: No Evidence of Benefit, Supported by Epidemiology and In Vitro Data, European Urology, doi:10.1016/j.eururo.2021.12.013.
Gedeborg et al., Androgen deprivation therapy, comorbidity, cancer stage and mortality from COVID-19 in men with prostate cancer, Scandinavian Journal of Urology, doi:10.1080/21681805.2021.2019304.
Lyon et al., 5α-Reductase Inhibitors Are Associated with Reduced Risk of SARS-CoV-2 Infection: A Matched-Pair, Registry-Based Analysis, Journal of Urology, doi:10.1097/JU.0000000000002180.
Lee et al., A population-level analysis of the protective effects of androgen deprivation therapy against COVID-19 disease incidence and severity, Frontiers in Medicine, doi:10.3389/fmed.2022.774773.
MacFadden (C) et al., Screening Large Population Health Databases for Potential COVID-19 Therapeutics: A Pharmacopeia-Wide Association Study (PWAS) of Commonly Prescribed Medications, Open Forum Infectious Diseases, doi:10.1093/ofid/ofac156.
Shah et al., The Impact of Androgen Deprivation Therapy on COVID-19 Illness in Men With Prostate Cancer, JNCI Cancer Spectrum, doi:10.1093/jncics/pkac035.
Cousins (B) et al., Integrative analysis of functional genomic screening and clinical data identifies a protective role for spironolactone in severe COVID-19, Cell Reports Methods, doi:10.1016/j.crmeth.2023.100503.
Davidsson et al., Androgen deprivation therapy in men with prostate cancer is not associated with COVID‐2019 infection, The Prostate, doi:10.1002/pros.24485.
Cousins (C) et al., Association between spironolactone use and COVID-19 outcomes in population-scale claims data: a retrospective cohort study, medRxiv, doi:10.1101/2023.02.28.23286515.
Valerio-Pascua et al., Chlorpheniramine Intranasal Spray to Accelerate COVID-19 Clinical Recovery in an Outpatient Setting: The ACCROS Trials, Research Square, doi:10.21203/rs.3.rs-2167465/v1.
Valerio-Pascua (B) et al., Chlorpheniramine Intranasal Spray to Accelerate COVID-19 Clinical Recovery in an Outpatient Setting: The ACCROS Trials, Research Square, doi:10.21203/rs.3.rs-2167465/v1.
Sanchez-Gonzalez et al., Intranasal Chlorpheniramine Maleate for the treatment of COVID-19: Translational and Clinical Evidence, Medical Research Archives, doi:10.18103/mra.v10i3.2752.
Klussmann et al., Early intervention with azelastine nasal spray may reduce viral load in SARS-CoV-2 infected patients, Scientific Reports, doi:10.1038/s41598-023-32546-z.
Mura et al., Real-world evidence for improved outcomes with histamine antagonists and aspirin in 22,560 COVID-19 patients, Signal Transduction and Targeted Therapy, doi:10.1038/s41392-021-00689-y.
Salvucci et al., Antihistamines improve cardiovascular manifestations and other symptoms of long-COVID attributed to mast cell activation, Frontiers in Cardiovascular Medicine, doi:10.3389/fcvm.2023.1202696.
Meiser et al., Azelastine Nasal Spray in Non-Hospitalized Subjects with Mild COVID-19 Infection: A Randomized Placebo-Controlled, Parallel-Group, Multicentric, Phase II Clinical Trial, Viruses, doi:10.3390/v16121914.
Vila‐Corcoles et al., Use of distinct anti‐hypertensive drugs and risk for COVID‐19 among hypertensive people: A population‐based cohort study in Southern Catalonia, Spain, The Journal of Clinical Hypertension, doi:10.1111/jch.13948.
Hoertel et al., Association between Hydroxyzine Use and Reduced Mortality in Patients Hospitalized for Coronavirus Disease 2019: Results from a multicenter observational study, medRxiv, doi:10.1101/2020.10.23.20154302.
Vila-Córcoles et al., Influence of prior comorbidities and chronic medications use on the risk of COVID-19 in adults: a population-based cohort study in Tarragona, Spain, BMJ Open, doi:10.1136/bmjopen-2020-041577.
Reznikov et al., Identification of antiviral antihistamines for COVID-19 repurposing, Biochemical and Biophysical Research Communications, doi:10.1016/j.bbrc.2020.11.095.
McKeigue et al., Relation of severe COVID-19 to polypharmacy and prescribing of psychotropic drugs: the REACT-SCOT case-control study, BMC Medicine, doi:10.1186/s12916-021-01907-8.
Sánchez-Rico et al., Hydroxyzine Use and Mortality in Patients Hospitalized for COVID-19: A Multicenter Observational Study, Journal of Clinical Medicine, doi:10.3390/jcm10245891.
Monserrat Villatoro et al., A Case-Control of Patients with COVID-19 to Explore the Association of Previous Hospitalisation Use of Medication on the Mortality of COVID-19 Disease: A Propensity Score Matching Analysis, Pharmaceuticals, doi:10.3390/ph15010078.
Hunt (C) et al., Medications Associated with Lower Mortality in a SARS-CoV-2 Positive Cohort of 26,508 Veterans, Journal of General Internal Medicine, doi:10.1007/s11606-022-07701-3.
Loucera (C) et al., Real-world evidence with a retrospective cohort of 15,968 COVID-19 hospitalized patients suggests 21 new effective treatments, Virology Journal, doi:10.1186/s12985-023-02195-9.
Hoertel (B) et al., Medications Modulating the Acid Sphingomyelinase/Ceramide System and 28-Day Mortality among Patients with SARS-CoV-2: An Observational Study, Pharmaceuticals, doi:10.3390/ph16081107.
Saber-Moghaddam et al., Oral nano-curcumin formulation efficacy in management of mild to moderate hospitalized coronavirus disease-19 patients: An open label nonrandomized clinical trial, Phytotherapy Research, doi:10.1002/ptr.7004
, doi:10.1002/ptr.7004.
Aldwihi et al., Patients’ Behavior Regarding Dietary or Herbal Supplements before and during COVID-19 in Saudi Arabia, International Journal of Environmental Research and Public Health, doi:10.3390/ijerph18105086.
Pawar et al., Oral Curcumin With Piperine as Adjuvant Therapy for the Treatment of COVID-19: A Randomized Clinical Trial, Frontiers in Pharmacology, doi:10.3389/fphar.2021.669362.
Ahmadi et al., Oral nano-curcumin formulation efficacy in the management of mild to moderate outpatient COVID-19: A randomized triple-blind placebo-controlled clinical trial, Food Science and Nutrition, doi:10.1002/fsn3.2226.
Sankhe et al., A prospective, multi center, single blind, randomized controlled study evaluating “AyurCoro3” as an adjuvant in the treatment of mild to moderate COVID, Journal of Ayurveda and Integrated Medical Sciences, doi:10.21760/jaims.6.4.6.
Majeed et al., A Randomized, Double-Blind, Placebo-Controlled Study to Assess the Efficacy and Safety of a Nutritional Supplement (ImmuActive) for COVID-19 Patients, Evidence-Based Complementary and Alternative Medicine, doi:10.1155/2021/8447545.
Khan (B) et al., Oral Co-Supplementation of Curcumin, Quercetin, and Vitamin D3 as an Adjuvant Therapy for Mild to Moderate Symptoms of COVID-19—Results From a Pilot Open-Label, Randomized Controlled Trial, Frontiers in Pharmacology, doi:10.3389/fphar.2022.898062.
Askari et al., The efficacy of curcumin-piperine co-supplementation on clinical symptoms, duration, severity, and inflammatory factors in COVID-19 outpatients: a randomized double-blind, placebo-controlled trial, Trials, doi:10.1186/s13063-022-06375-w.
Chitre et al., Phase III randomized clinical trial of BV-4051, an Ayurvedic polyherbal formulation in moderate SARS-CoV-2 infections and its impact on inflammatory biomarkers, Phytotherapy Research, doi:10.1002/ptr.7683.
Din Ujjan et al., The possible therapeutic role of curcumin and quercetin in the early-stage of COVID-19—Results from a pragmatic randomized clinical trial, Frontiers in Nutrition, doi:10.3389/fnut.2022.1023997.
Kishimoto et al., Efficacy of highly bioavailable oral curcumin in asymptomatic or mild COVID-19 patients: a double-blind, randomized, placebo-controlled trial, Journal of Health, Population and Nutrition, doi:10.1186/s41043-024-00584-6.
Valizadeh et al., Nano-curcumin therapy, a promising method in modulating inflammatory cytokines in COVID-19 patients, Int. Immunopharmacol., doi:10.1016/j.intimp.2020.107088.
Tahmasebi et al., Nanocurcumin improves Treg cell responses in patients with mild and severe SARS-CoV2, Life Sciences, doi:10.1016/j.lfs.2021.119437.
Hassaniazad et al., A triple-blind, placebo-controlled, randomized clinical trial to evaluate the effect of curcumin-containing nanomicelles on cellular immune responses subtypes and clinical outcome in COVID-19 patients, Phytotherapy Research, doi:10.1002/ptr.7294.
Asadirad et al., Antiinflammatory potential of nano-curcumin as an alternative therapeutic agent for the treatment of mild-to-moderate hospitalized COVID-19 patients in a placebo-controlled clinical trial, Phytotherapy Research, doi:10.1002/ptr.7375.
Kartika et al., Curcumin as adjuvant Therapy in Mild - Moderate Covid 19, ICE on IMERI, 2021, web.archive.org/web/20220209083251/http://writingcenter.fk.ui.ac.id/index.php/p/article/view/40.
Hartono et al., The Effect of Curcumin and Virgin Coconut Oil Towards Cytokines Levels in COVID-19 Patients at Universitas Sebelas Maret Hospital, Surakarta, Indonesia, Pharmacognosy Journal, doi:10.5530/pj.2022.14.27.
Thomas et al., A Randomised, Double-Blind, Placebo-Controlled Trial Evaluating Concentrated Phytochemical-Rich Nutritional Capsule in Addition to a Probiotic Capsule on Clinical Outcomes among Individuals with COVID-19—The UK Phyto-V Study, COVID, doi:10.3390/covid2040031.
Sankhe (B) et al., A Randomized, Controlled, Blinded, Parallel Group, Clinical Trial to study the role of Ayurcov (AyurCoro3), one day regimen as an adjuvant therapy for COVID-19 disease management, at dedicated Covid Hospital (DCH) in India, Complementary Therapies in Medicine, doi:10.1016/j.ctim.2022.102824.
Hellou et al., Effect of ArtemiC in patients with COVID-19: A Phase II prospective study, Journal of Cellular and Molecular Medicine, doi:10.1111/jcmm.17337.
Abbaspour-Aghdam et al., Immunomodulatory role of Nanocurcumin in COVID-19 patients with dropped natural killer cells frequency and function, European Journal of Pharmacology, doi:10.1016/j.ejphar.2022.175267.
Sadeghizadeh et al., Promising clinical outcomes of nano‐curcumin treatment as an adjunct therapy in hospitalized COVID‐19 patients: A randomized, double‐blinded, placebo‐controlled trial, Phytotherapy Research, doi:10.1002/ptr.7844.
Gérain et al., NASAFYTOL® supplementation in adults hospitalized with COVID-19 infection: results from an exploratory open-label randomized controlled trial, Frontiers in Nutrition, doi:10.3389/fnut.2023.1137407.
Ahmadi (B) et al., Efficacy of Nanocurcumin as an Add-On Treatment for Patients Hospitalized with COVID-19: A Double-Blind, Randomized Clinical Trial, International Journal of Clinical Practice, doi:10.1155/2023/5734675.
Bejan et al., DrugWAS: Drug‐wide Association Studies for COVID‐19 Drug Repurposing, Clinical Pharmacology & Therapeutics, doi:10.1002/cpt.2376.
Shehab et al., Immune-boosting effect of natural remedies and supplements on progress of, and recovery from COVID-19 infection, Tropical Journal of Pharmaceutical Research, doi:10.4314/tjpr.v21i2.13.
Nimer et al., Effect of natural products use prior to infection with COVID-19 on disease severity and hospitalization: A self-reported cross-sectional survey study, F1000Research, doi:10.12688/f1000research.121933.1.
Lissoni et al., COVID-19 Disease as an Acute Angiotensin 1-7 Deficiency: A Preliminary Phase 2 Study with Angiotensin 1-7 in Association with Melatonin and Cannabidiol in Symptomatic COVID19 -Infected Subjects, Journal of Infectiology, doi:10.29245/2689-9981/2018/2.1162.
Alizadeh et al., A Pilot Study on Controlling Coronavirus Disease 2019 (COVID-19) Inflammation Using Melatonin Supplement, Iranian Journal of Allergy, Asthma and Immunology, ijaai.tums.ac.ir/index.php/ijaai/article/view/3086.
Ramlall et al., Melatonin is significantly associated with survival of intubated COVID-19 patients, medRxiv, doi:10.1101/2020.10.15.20213546.
Darban et al., Efficacy of High Dose Vitamin C, Melatonin and Zinc in Iranian Patients with Acute Respiratory Syndrome due to Coronavirus Infection: A Pilot Randomized Trial, Journal of Cellular & Molecular Anesthesia, doi:10.22037/jcma.v6i2.32182.
Hosseini et al., Evaluation of Th1 and Th2 mediated cellular and humoral immunity in patients with COVID-19 following the use of melatonin as an adjunctive treatment, European Journal of Pharmacology, doi:10.1016/j.ejphar.2021.174193.
Farnoosh et al., Efficacy of a Low Dose of Melatonin as an Adjunctive Therapy in Hospitalized Patients with COVID-19: A Randomized, Double-blind Clinical Trial, Archives of Medical Research, doi:10.1016/j.arcmed.2021.06.006.
Sánchez-González, What if melatonin could help COVID-19 severe patients?, jcsm.aasm.org/doi/10.5664/jcsm.9554.
Mousavi et al., Melatonin effects on sleep quality and outcomes of COVID-19 patients: An open-label, randomized, controlled trial, Journal of Medical Virology, doi:10.1002/jmv.27312.
Hasan et al., The Effect of Melatonin on Thrombosis, Sepsis and Mortality Rate in COVID-19 Patients, International Journal of Infectious Diseases, doi:10.1016/j.ijid.2021.10.012.
Bologna et al., Efficacy of Prolonged-Release Melatonin 2 mg (PRM 2 mg) Prescribed for Insomnia in Hospitalized Patients for COVID-19: A Retrospective Observational Study, Journal of Clinical Medicine, doi:10.3390/jcm10245857.
Sánchez-Rico (B) et al., Melatonin does not reduce mortality in adult hospitalized patients with COVID-19: a multicenter retrospective observational study, Journal of Travel Medicine, doi:10.1093/jtm/taab195.
Karimpour-razkenari et al., Melatonin as adjunctive therapy in patients admitted to the Covid-19, Annals of Medicine and Surgery, doi:10.1016/j.amsu.2022.103492.
Alizadeh (B) et al., High dose melatonin as an adjuvant therapy in intubated patients with COVID-19: A randomized clinical trial, Journal of Taibah University Medical Sciences, doi:10.1016/j.jtumed.2022.04.012.
Fogleman et al., A Pilot of a Randomized Control Trial of Melatonin and Vitamin C for Mild-to-Moderate COVID-19, The Journal of the American Board of Family Medicine, doi:10.3122/jabfm.2022.04.210529.
Ameri et al., Efficacy and safety of oral melatonin in patients with severe COVID-19: a randomized controlled trial, Inflammopharmacology, doi:10.1007/s10787-022-01096-7.
Jehi et al., Individualizing Risk Prediction for Positive Coronavirus Disease 2019 Testing, Chest, doi:10.1016/j.chest.2020.05.580.
Zhou et al., A network medicine approach to investigation and population-based validation of disease manifestations and drug repurposing for COVID-19, PLOS Biology, doi:10.1371/journal.pbio.3000970.
García-García et al., Melatonin in the Prophylaxis of SARS-CoV-2 Infection in Healthcare Workers (MeCOVID): A Randomised Clinical Trial, Journal of Clinical Medicine, doi:10.3390/jcm11041139.
Hunt (D) et al., Medications Associated with Lower Mortality in a SARS-CoV-2 Positive Cohort of 26,508 Veterans, Journal of General Internal Medicine, doi:10.1007/s11606-022-07701-3.
Hassan et al., The effects of probiotic Lactobacillus acidophilus and colchicine on the control of symptoms, duration, and disease progression of mild and moderate cases of COVID-19: A randomized controlled clinical trial, Research Square, doi:10.21203/rs.3.rs-3049708/v1.
Inokuchi et al., Oral Colchicine and Low-Dose Aspirin Combination Therapy for Non-elderly, Non-severe, Early Time From Onset, Adult Outpatients with Coronavirus Disease 2019 (COVID-19) during “The Fifth Pandemic Wave” in Japan, The Kurume Medical Journal, doi:10.2739/kurumemedj.MS7012003.
Deftereos et al., Effect of Colchicine vs Standard Care on Cardiac and Inflammatory Biomarkers and Clinical Outcomes in Patients Hospitalized With Coronavirus Disease 2019: The GRECCO-19 Randomized Clinical Trial, JAMA Network Open, doi:10.1001/jamanetworkopen.2020.13136.
Lopes et al., Beneficial effects of colchicine for moderate to severe COVID-19: a randomised, double-blinded, placebo-controlled clinical trial, RMD Open, doi:10.1136/rmdopen-2020-001455.
Brunetti et al., Colchicine to Weather the Cytokine Storm in Hospitalized Patients with COVID-19, Journal of Clinical Medicine, doi:10.3390/jcm9092961.
Scarsi et al., Association between treatment with colchicine and improved survival in a single-centre cohort of adult hospitalised patients with COVID-19 pneumonia and acute respiratory distress syndrome, Annals of the Rheumatic Diseases, doi:10.1136/annrheumdis-2020-217712.
Salehzadeh et al., The Impact of Colchicine on COVID-19 patients: A Clinical Trial Study, Mediterranean Journal of Rheumatology, doi:10.31138/mjr.33.2.232.
Pinzón et al., Clinical Outcome of Patients with COVID-19 Pneumonia Treated with Corticosteroids and Colchicine in Colombia, Research Square, doi:10.21203/rs.3.rs-94922/v1.
Sandhu (B) et al., A Case Control Study to Evaluate the Impact of Colchicine on Patients Admitted to the Hospital with Moderate to Severe COVID-19 Infection, Canadian Journal of Infectious Diseases and Medical Microbiology, doi:10.1155/2020/8865954.
Mahale (B) et al., A Retrospective Observational Study of Hypoxic COVID-19 Patients Treated with Immunomodulatory Drugs in a Tertiary Care Hospital, Indian Journal of Critical Care Medicine, doi:10.5005/jp-journals-10071-23599.
Valerio Pascua et al., A multi-mechanism approach reduces length of stay in the ICU for severe COVID-19 patients, PLOS ONE, doi:10.1371/journal.pone.0245025.
Tardif et al., Colchicine for community-treated patients with COVID-19 (COLCORONA): a phase 3, randomised, double-blinded, adaptive, placebo-controlled, multicentre trial, The Lancet Respiratory Medicine, doi:10.1016/S2213-2600(21)00222-8.
Mareev (B) et al., Proactive anti-inflammatory therapy with colchicine in the treatment of advanced stages of new coronavirus infection. The first results of the COLORIT study, Kardiologiia, doi:10.18087/cardio.2021.2.n1560.
García-Posada et al., Clinical outcomes of patients hospitalized for COVID-19 and evidence-based on the pharmacological management reduce mortality in a region of the Colombian Caribbean, Journal of Infection and Public Health, doi:10.1016/j.jiph.2021.02.013.
Manenti et al., Reduced mortality in COVID-19 patients treated with colchicine: Results from a retrospective, observational study, PLOS ONE, doi:10.1371/journal.pone.0248276.
Mostafaie et al., Colchicine Plus Phenolic Monoterpenes to Treat COVID-19, NCT04392141, clinicaltrials.gov/ct2/show/NCT04392141.
Recovery Collaborative Group, Colchicine in patients admitted to hospital with COVID-19 (RECOVERY): a randomised, controlled, open-label, platform trial, The Lancet Respiratory Medicine, doi:10.1016/S2213-2600(21)00435-5.
Hueda-Zavaleta et al., Factores asociados a la muerte por COVID-19 en pacientes admitidos en un hospital público en Tacna, Perú, Revista Peruana de Medicina Experimental y Salud Pública, doi:10.17843/rpmesp.2021.382.7158.
Kevorkian et al., Oral corticoid, aspirin, anticoagulant, colchicine, and furosemide to improve the outcome of hospitalized COVID-19 patients - the COCAA-COLA cohort study, Journal of Infection, doi:10.1016/j.jinf.2021.02.008.
Gaitán-Duarte et al., Effectiveness of rosuvastatin plus colchicine, emtricitabine/tenofovir and combinations thereof in hospitalized patients with COVID-19: a pragmatic, open-label randomized trial, eClinicalMedicine, doi:10.1016/j.eclinm.2021.101242.
Pascual-Figal et al., Colchicine in Recently Hospitalized Patients with COVID-19: A Randomized Controlled Trial (COL-COVID), International Journal of General Medicine, doi:10.2147/IJGM.S329810.
Dorward et al., Colchicine for COVID-19 in the community (PRINCIPLE): a randomised, controlled, adaptive platform trial, British Journal of General Practice, doi:10.3399/BJGP.2022.0083.
Absalón-Aguilar et al., Colchicine Is Safe Though Ineffective in the Treatment of Severe COVID-19: a Randomized Clinical Trial (COLCHIVID), Journal of General Internal Medicine, doi:10.1007/s11606-021-07203-8.
Diaz et al., Effect of Colchicine vs Usual Care Alone on Intubation and 28-Day Mortality in Patients Hospitalized With COVID-19, JAMA Network Open, doi:10.1001/jamanetworkopen.2021.41328.
Alsultan et al., Efficacy of Colchicine and Budesonide in Improvement Outcomes of Patients with Coronavirus Infection 2019 in Damascus, Syria: A Randomized Control Trial, Interdisciplinary Perspectives on Infectious Diseases, doi:10.1155/2021/2129006.
Karakaş et al., Reducing length of hospital stay with colchicine, The Journal of Infection in Developing Countries, doi:10.3855/jidc.14924.
Pourdowlat et al., Efficacy and safety of colchicine treatment in patients with COVID-19: A prospective, multicenter, randomized clinical trial, Phytotherapy Research, doi:10.1002/ptr.7319.
Gorial (B) et al., Randomized controlled trial of colchicine add on to the standard therapy in moderate and severe corona virus Disease-19 infection, Annals of Medicine and Surgery, doi:10.1016/j.amsu.2022.103593.
Pimenta Bonifácio et al., Efficacy and safety of Ixekizumab vs. low-dose IL-2 vs. Colchicine vs. standard of care in the treatment of patients hospitalized with moderate-to-critical COVID-19: A pilot randomized clinical trial (STRUCK: Survival Trial Using Cytokine Inhibitors), Revista da Sociedade Brasileira de Medicina Tropical, doi:10.1590/0037-8682-0565-2022.
Jalal et al., Effectiveness of colchicine among patients with COVID-19 infection: A randomized, open-labeled, clinical trial, Indian Journal of Rheumatology, doi:10.4103/injr.injr_264_21.
Cecconi et al., Efficacy of short-course colchicine treatment in hospitalized patients with moderate to severe COVID-19 pneumonia and hyperinflammation: a randomized clinical trial, Scientific Reports, doi:10.1038/s41598-022-13424-6.
Eikelboom et al., Colchicine and the combination of rivaroxaban and aspirin in patients hospitalised with COVID-19 (ACT): an open-label, factorial, randomised, controlled trial, The Lancet Respiratory Medicine, doi:10.1016/S2213-2600(22)00298-3.
Eikelboom (B) et al., Colchicine and aspirin in community patients with COVID-19 (ACT): an open-label, factorial, randomised, controlled trial, The Lancet Respiratory Medicine, doi:10.1016/S2213-2600(22)00299-5.
Perricone et al., Treatment with COLchicine in hospitalized patients affected by COVID-19: the COLVID-19 trial, European Journal of Internal Medicine, doi:10.1016/j.ejim.2022.10.016.
Rahman et al., Efficacy of colchicine in patients with moderate COVID-19: A double-blinded, randomized, placebo-controlled trial, PLOS ONE, doi:10.1371/journal.pone.0277790.
Hueda-Zavaleta (B) et al., Determination of PaO2/FiO2 after 24 h of invasive mechanical ventilation and ΔPaO2/FiO2 at 24 h as predictors of survival in patients diagnosed with ARDS due to COVID-19, PeerJ, doi:10.7717/peerj.14290.
Haroon et al., Colchicine anti-inflammatory therapy for non-intensive care unit hospitalized COVID-19 patients: results from a pilot open-label, randomized controlled clinical trial, Journal of Physiology and Pharmacology, doi:10.26402/jpp.2022.3.09.
Kasiri et al., The effects of colchicine on hospitalized COVID-19 patients: A randomized, double-blind, placebo-controlled clinical trial, Journal of Investigative Medicine, doi:10.1177/10815589221141815.
Sunil Naik et al., Effect of colchicine and aspirin given together in patients with moderate COVID-19, Contemporary Clinical Trials Communications, doi:10.1016/j.conctc.2023.101070.
Shah (B) et al., Colchicine and high-intensity rosuvastatin in the treatment of non-critically ill patients hospitalised with COVID-19: a randomised clinical trial, BMJ Open, doi:10.1136/bmjopen-2022-067910.
Villamañán et al., Targeting patients with pneumonia by COVID-19 that could be beneficiated by colchicine, Eur. J. Hosp. Pharm., doi:10.1136/ejhpharm-2023-eahp.56.
Yadollahzadeh et al., Colchicine with Infliximab Compared to Infliximab in Hospitalized Patients with COVID-19 Pneumonia: An Open-label Randomized Trial, Coronaviruses, doi:10.2174/0126667975271636231109051950.
Vaziri et al., Investigating efficacy of colchicine plus phenolic monoterpenes fraction as a potential treatment for patients diagnosed with COVID-19: A randomized controlled parallel clinical trial, Heliyon, doi:10.1016/j.heliyon.2024.e27373.
Gertner et al., Colchicine and/or Naltrexone for Hospitalized COVID-19 Patients Not Requiring High Levels of Ventilatory Support (COLTREXONE): A Prospective, Randomized, Open-Label Trial, Cureus, doi:10.7759/cureus.60364.
Landi et al., Edoxaban and/or colchicine for patients with coronavirus disease 2019 managed in the out-of-hospital setting (CONVINCE): a randomized clinical trial, Journal of Cardiovascular Medicine, doi:10.2459/JCM.0000000000001639.
Madrid-García et al., Influence of colchicine prescription in COVID-19-related hospital admissions: a survival analysis, Therapeutic Advances in Musculoskeletal Disease, doi:10.1177/1759720x211002684.
Ozcifci et al., The incidence, clinical characteristics, and outcome of COVID-19 in a prospectively followed cohort of patients with Behçet’s syndrome, Rheumatology International, doi:10.1007/s00296-021-05056-2.
Monserrat Villatoro (B) et al., A Case-Control of Patients with COVID-19 to Explore the Association of Previous Hospitalisation Use of Medication on the Mortality of COVID-19 Disease: A Propensity Score Matching Analysis, Pharmaceuticals, doi:10.3390/ph15010078.
Topless et al., Gout and the risk of COVID-19 diagnosis and death in the UK Biobank: a population-based study, The Lancet Rheumatology, doi:10.1016/S2665-9913(21)00401-X.
Oztas (B) et al., Frequency and Severity of COVID-19 in Patients with Various Rheumatic Diseases Treated Regularly with Colchicine or Hydroxychloroquine, Journal of Medical Virology, doi:10.1002/jmv.27731.
Avanoglu Guler et al., COVID-19 in familial Mediterranean fever: Clinical course and complications related to primary disease, Modern Rheumatology, doi:10.1093/mr/roac074.
Correa-Rodríguez et al., Clinical course of Covid-19 in a cohort of patients with Behçet disease, Medicina Clínica (English Edition), doi:10.1016/j.medcle.2022.08.009.
Sáenz-Aldea et al., Colchicine and risk of hospitalisation due to COVID-19: a population-based study, Journal of Medical Virology, doi:10.1002/jmv.28496.
Chevalier (B) et al., CovAID: Identification of factors associated with severe COVID-19 in patients with inflammatory rheumatism or autoimmune diseases, Frontiers in Medicine, doi:10.3389/fmed.2023.1152587.
Haran et al., Targeting the Microbiome With KB109 in Outpatients with Mild to Moderate COVID-19 Reduced Medically Attended Acute Care Visits and Improved Symptom Duration in Patients With Comorbidities, medRxiv, doi:10.1101/2021.03.26.21254422.
Gutiérrez-Castrellón et al., Probiotic improves symptomatic and viral clearance in Covid19 outpatients: a randomized, quadruple-blinded, placebo-controlled trial, Gut Microbes, doi:10.1080/19490976.2021.2018899.
Veterini et al., Probiotics Intake as Adjunct Therapy for Infected Health-Care with SARS COV-2, Indian Journal of Forensic Medicine & Toxicology, 15:2, medicopublication.com/index.php/ijfmt/article/view/15003/13584.
Hassan (B) et al., The effects of probiotic Lactobacillus acidophilus and colchicine on the control of symptoms, duration, and disease progression of mild and moderate cases of COVID-19: A randomized controlled clinical trial, Research Square, doi:10.21203/rs.3.rs-3049708/v1.
Kolesnyk et al., The role of nutritional support with probiotics in outpatients with symptomatic acute respiratory tract infections: a multicenter, randomized, double-blind, placebo-controlled dietary study, BMC Nutrition, doi:10.1186/s40795-023-00816-8.
d'Ettorre et al., Challenges in the Management of SARS-CoV2 Infection: The Role of Oral Bacteriotherapy as Complementary Therapeutic Strategy to Avoid the Progression of COVID-19, Frontiers in Medicine, doi:10.3389/fmed.2020.00389.
Ceccarelli et al., Oral Bacteriotherapy in Patients With COVID-19: A Retrospective Cohort Study, Frontiers in Medicine, doi:10.3389/fnut.2020.613928.
Shah (C) et al., Potential of the Combination of a Systemic Enzyme Complex and Probiotics administration to Combat COVID-19: A Randomized Open Label Prospective Analysis, Advances in Clinical Toxicology, doi:10.23880/act-16000204.
Li (E) et al., The role of probiotics in coronavirus disease-19 infection in Wuhan: A retrospective study of 311 severe patients, International Immunopharmacology, doi:10.1016/j.intimp.2021.107531.
Zhang et al., Probiotics use is associated with improved clinical outcomes among hospitalized patients with COVID-19, Therapeutic Advances in Gastroenterology
, doi:10.1177/17562848211035670.
Ceccarelli (B) et al., Oxygen Sparing Effect of Bacteriotherapy in COVID-19, Nutrients, doi:10.3390/nu13082898.
Ivashkin et al., Efficacy of a Probiotic Consisting of Lacticaseibacillus rhamnosus PDV 1705, Bifidobacterium bifidum PDV 0903, Bifidobacterium longum subsp. infantis PDV 1911, and Bifidobacterium longum subsp. longum PDV 2301 in the Treatment of Hospitalized Patients with COVID-19: a Randomized Controlled Trial, Probiotics Antimicrob Proteins, doi:10.1007/s12602-021-09858-5.
Zhang (B) et al., Gut microbiota-derived synbiotic formula (SIM01) as a novel adjuvant therapy for COVID-19: An open-label pilot study, Journal of Gastroenterology and Hepatology, doi:10.1111/jgh.15796.
Saviano et al., COVID-19 Pneumonia and Gut Inflammation: The Role of a Mix of Three Probiotic Strains in Reducing Inflammatory Markers and Need for Oxygen Support, Journal of Clinical Medicine, doi:10.3390/jcm11133758.
Trinchieri et al., Exploiting Bacteria for Improving Hypoxemia of COVID-19 Patients, Biomedicines, doi:10.3390/biomedicines10081851.
Di Pierro et al., Clinical Effects of Streptococcus salivarius K12 in Hospitalized COVID-19 Patients: Results of a Preliminary Study, Microorganisms, doi:10.3390/microorganisms10101926.
Giancola et al., Efficacy of a Multistrain Synbiotic Treatment in Acute and Post-Acute COVID-19 Patients: A Double-Blind, Placebo-Controlled Randomized Trial, Microorganisms, doi:10.3390/microorganisms12071443.
Louca et al., Modest effects of dietary supplements during the COVID-19 pandemic: insights from 445 850 users of the COVID-19 Symptom Study app, BMJ Nutrition, Prevention & Health, doi:10.1136/bmjnph-2021-000250.
Di Pierro (B) et al., The administration of S. salivarius K12 to children may reduce the rate of SARS-CoV-2 infection, Minerva Medica, doi:10.23736/S0026-4806.21.07487-5.
Holt (C) et al., Risk factors for developing COVID-19: a population-based longitudinal study (COVIDENCE UK), Thorax, doi:10.1136/thoraxjnl-2021-217487.
Ahanchian et al., Synbiotic for Prevention of SARS-Cov2 Infection in High Risk Hospital Staffs: A Randomized Controlled Trial, Open Journal of Nursing, doi:10.4236/ojn.2021.115025.
Fernández-Ferreiro et al., Effects of Loigolactobacillus coryniformis K8 CECT 5711 on the Immune Response of Elderly Subjects to COVID-19 Vaccination: A Randomized Controlled Trial, Nutrients, doi:10.3390/nu14010228.
Wischmeyer et al., Daily Lactobacillus Probiotic versus Placebo in COVID-19-Exposed Household Contacts (PROTECT-EHC): A Randomized Clinical Trial, medRxiv, doi:10.1101/2022.01.04.21268275.
Rodriguez-Blanque et al., Evaluation of the effect of Loigolactobacillus coryniformis K8 CECT 5711 consumption in health care workers exposed to COVID-19, Frontiers in Nutrition, doi:10.3389/fnut.2022.962566.
Catinean et al., Ongoing Treatment with a Spore-Based Probiotic Containing Five Strains of Bacillus Improves Outcomes of Mild COVID-19, Nutrients, doi:10.3390/nu15030488.
Di Pierro (C) et al., Role of S. salivarius K12 in the prevention of URTI and AGE in nursery-aged children, Minerva Medica, doi:10.23736/S0026-4806.23.08920-6.
Sarlin et al., Streptococcus salivarius Probiotics to Prevent Acute Otitis Media in Children, JAMA Network Open, doi:10.1001/jamanetworkopen.2023.40608.
Chen (H) et al., Oral Azvudine (FNC) Tablets in Patients infected with SARS-CoV-2 Omicron Variant: A Retrospective Cohort Study, medRxiv, doi:10.1101/2023.01.05.23284180.
Han et al., Effectiveness and Optimal Timing of Azvudine in COVID-19 Patients: A Multi-center Retrospective Study in Beijing, China, Research Square, doi:10.21203/rs.3.rs-3145554/v1.
Yang et al., Oral azvudine for mild‐to‐moderate COVID‐19 in high risk, nonhospitalized adults: Results of a real‐world study, Journal of Medical Virology, doi:10.1002/jmv.28947.
Wang (D) et al., Antiviral effectiveness and survival correlation of azvudine and nirmatrelvir/ritonavir in elderly severe patients with COVID-19: a retrospective real-world study, eClinicalMedicine, doi:10.1016/j.eclinm.2024.102468.
Jin et al., Effects of Azvudine on the Low-Risk Patients Infected with COVID-19 Omicron Variants: A Retrospective Case-Control Study, Journal of Clinical Pharmacology and Therapeutics, 5:1, www.medtextpublications.com/open-access/effects-of-azvudine-on-the-low-risk-patients-infected-with-covid-19-1584.pdf.
Wu (B) et al., Azvudine for the Treatment of COVID‐19 in Pre‐Existing Cardiovascular Diseases: A Single‐Center, Real‐World Experience, Advanced Science, doi:10.1002/advs.202306050.
Zou et al., Risk prediction and early intervention strategies for persistent SARS-CoV-2 infection in patients with non-Hodgkin lymphoma: a retrospective cohort study, BMC Pulmonary Medicine, doi:10.1186/s12890-025-03524-0.
Shen et al., Real-world effectiveness of Azvudine in hospitalized patients with COVID-19: a retrospective cohort study, medRxiv, doi:10.1101/2023.01.23.23284899.
Chen (I) et al., All-cause mortality in moderate and severe COVID-19 patients with myocardial injury receiving versus not receiving azvudine: a propensity score-matched analysis, Cardiology Plus, doi:10.1097/CP9.0000000000000049.
Sun et al., Oral Azvudine for hospitalised patients with COVID-19 and pre-existing conditions: a retrospective cohort study, eClinicalMedicine, doi:10.1016/j.eclinm.2023.101981.
Zong et al., Azvudine reduces the in-hospital mortality of COVID-19 patients: A retrospective cohort study, Acta Pharmaceutica Sinica B, doi:10.1016/j.apsb.2023.07.007.
Shao et al., Composite Interventions on Outcomes of Severely and Critically Ill Patients with COVID-19 in Shanghai, China, Microorganisms, doi:10.3390/microorganisms11071859.
Dian et al., Azvudine versus Paxlovid for oral treatment of COVID-19 in Chinese patients with pre-existing comorbidities, Journal of Infection, doi:10.1016/j.jinf.2023.05.012.
Zhou (B) et al., Secondary pulmonary infection and co-infection in elderly COVID-19 patients during the pandemics in a tertiary general hospital in Beijing, China, Frontiers in Microbiology, doi:10.3389/fmicb.2023.1280026.
Wei et al., Head-to-head comparison of azvudine and nirmatrelvir/ritonavir for the hospitalized patients with COVID-19: a real-world retrospective cohort study with propensity score matching, Frontiers in Pharmacology, doi:10.3389/fphar.2023.1274294.
de Souza et al., Phase III, randomized, double-blind, placebo-controlled clinical study: a study on the safety and clinical efficacy of AZVUDINE in moderate COVID-19 patients, Frontiers in Medicine, doi:10.3389/fmed.2023.1215916.
Liu (B) et al., Clinical characteristics, outcomes, and risk factors of SARS-CoV-2 breakthrough infections among 572 fully vaccinated (BBIBP-CorV) hospitalized patients, Heliyon, doi:10.1016/j.heliyon.2023.e21387.
Li (F) et al., Risk of severe case in COVID-19 patients and Azvudine: A Retrospective cohort study after exit from ‘zero-COVID’ policy, Research Square, doi:10.21203/rs.3.rs-3707560/v1.
Peng (B) et al., Impact of Anti-angiogenic Drugs on Severity of COVID-19 in Patients with Non-Small Cell Lung Cancer, Technology in Cancer Research & Treatment, doi:10.1177/15330338241248573.
Li (G) et al., A Retrospective Analysis of Azvudine in Patients with COVID-19 and Pre-existing Cancer, Journal of Cancer, doi:10.7150/jca.91530.
Zhong (B) et al., Azvudine reduces the mortality rate of patients with Coronavirus disease 2019: a single-center retrospective analysis study, Research Square, doi:10.21203/rs.3.rs-4157424/v1.
Liu (C) et al., Effectiveness of Azvudine and Nirmatrelvir-ritonavir in Kidney Transplant Recipients With COVID-19: A Retrospective Cohort Study, Infections in the immunosuppressed and immunocompromised host, doi:10.1164/ajrccm-conference.2024.209.1_MeetingAbstracts.A2917.
Lv (B) et al., Clinical characteristics and outcomes of hospitalized kidney transplant recipients with COVID-19 infection in China during the Omicron wave: a single-center cohort study, Journal of Zhejiang University - SCIENCE B (Biomedicine & Biotechnology, doi:10.1631/jzus.B2300538.
Zhang (C) et al., Efficacy of Azvudine Therapy in Patients with Severe and Non-Severe COVID‐19: A Propensity Score-Matched Analysis, Infection and Drug Resistance, doi:10.2147/IDR.S481591.
Xu (B) et al., Systematic evaluation of therapeutic effectiveness of Azvudine in treating COVID-19 hospitalized patients: a retrospective cohort study, Frontiers in Cellular and Infection Microbiology, doi:10.3389/fcimb.2024.1453234.
Ren et al., Real-world effectiveness and safety of Azvudine in hospitalized patients with SARS-CoV-2 infection: a multicenter, retrospective cohort study, Journal of Infection, doi:10.1016/j.jinf.2024.106355.
Zhang (D) et al., Efficacy of azvudine plus dexamethasone in severe hospitalized patients with Omicron infection: a prospective multicenter study, Frontiers in Cellular and Infection Microbiology, doi:10.3389/fcimb.2024.1390098.
Zhu et al., Real-world efficacy and safety of azvudine in hospitalized older patients with COVID-19 during the omicron wave in China: A retrospective cohort study, Acta Pharmaceutica Sinica B, doi:10.1016/j.apsb.2024.12.004.
Yuan et al., Characteristics of patients with non-severe infections of different SARS-CoV-2 omicron subvariants in China, Frontiers in Medicine, doi:10.3389/fmed.2024.1511227.
Zhong (C) et al., Azvudine efficacy in reducing mortality in COVID-19 patients, European Journal of Medical Research, doi:10.1186/s40001-024-02220-9.
Sun (B) et al., Antiviral effectiveness and safety of azvudine in hospitalized SARS‐CoV‐2 patients with pre‐existing chronic respiratory diseases: A multicenter, retrospective cohort study, VIEW, doi:10.1002/VIW.20240133.
Zhou (C) et al., A multicenter, real-world cohort study: effectiveness and safety of Azvudine in hospitalized COVID-19 patients with pre-existing diabetes, Frontiers in Endocrinology, doi:10.3389/fendo.2025.1467303.
He (C) et al., Prognostic factors in hospitalized patients with COVID-19 pneumonia and effectiveness of prophylactic anticoagulant therapy: a single-center retrospective study, BMC Infectious Diseases, doi:10.1186/s12879-025-10666-3.
Yu (D) et al., The effectiveness and safety of azvudine treatment in COVID‐19 patients with kidney disease based on a multicenter retrospective cohort study, VIEW, doi:10.1002/VIW.20240075.
Altay et al., Combined Metabolic Activators Accelerates Recovery in Mild-to-Moderate COVID-19, Advanced Science, doi:10.1002/advs.202101222.
Ignatova et al., Therapeutic possibilities of using an expectorant mucolytic agent with antioxidant properties in COVID-19 infection, Russian Medical Inquiry, doi:10.32364/2587-6821-2021-5-7-473-478.
de Alencar et al., Double-blind, Randomized, Placebo-controlled Trial With N-acetylcysteine for Treatment of Severe Acute Respiratory Syndrome Caused by Coronavirus Disease 2019 (COVID-19), Clinical Infectious Diseases, doi:10.1093/cid/ciaa1443.
Gaynitdinova et al., N-acetylcysteine as a part of complex treatment of moderate COVID-associated pneumonia, Pulmonologiya, doi:10.18093/0869-0189-2021-31-1-21-29.
Pellegrini et al., A Retrospective Analysis of Outcomes Amongst COVID-19 Infected Patients with Acute Hepatitis Receiving N-Acetylcysteine Therapy in a Safety Net Hospital, Gastroenterology, doi:10.1016/S0016-5085(21)02756-6.
Pourhoseingholi et al., Case Characteristics, Clinical Data, And Outcomes of Hospitalized COVID-19 Patients In Qom Province, Iran: A Prospective Cohort Study, Research Square, doi:10.21203/rs.3.rs-365321/v2.
Taher et al., A pilot study on intravenous N-Acetylcysteine treatment in patients with mild-to-moderate COVID19-associated acute respiratory distress syndrome, Pharmacological Reports, doi:10.1007/s43440-021-00296-2.
Assimakopoulos et al., N-acetyl-cysteine reduces the risk for mechanical ventilation and mortality in patients with COVID-19 pneumonia: a two-center retrospective cohort study, Infectious Diseases, doi:10.1080/23744235.2021.1945675.
Avdeev et al., N-acetylcysteine for the treatment of COVID-19 among hospitalized patients, Journal of Infection, doi:10.1016/j.jinf.2021.07.003.
Faverio et al., Impact of N-acetyl-l-cysteine on SARS-CoV-2 pneumonia and its sequelae: results from a large cohort study, ERJ Open Research, doi:10.1183/23120541.00542-2021.
Ramadhan et al., The Effects of N-Acetylcysteine as Adjuvant Therapy To Reduce TNF-Α Level And Increase SPO2/FIO2 Ratio In Improving Hypoxemia In COVID-19 Patients, Indonesian Journal of Tropical and Infectious Disease, 9:3, www.e-journal.unair.ac.id/IJTID/article/view/30874.
Izquierdo et al., Use of N-Acetylcysteine at high doses as an oral treatment for patients hospitalized with COVID-19, Science Progress, doi:10.1177/00368504221074574.
Delić et al., Effects of Different Inhalation Therapy on Ventilator-Associated Pneumonia in Ventilated COVID-19 Patients: A Randomized Controlled Trial, Microorganisms, doi:10.3390/microorganisms10061118.
Fariña-González et al., Hourly Analysis of Mechanical Ventilation Parameters in Critically Ill Adult Covid-19 Patients: Association with Mortality, Journal of Intensive Care Medicine, doi:10.1177/08850666221105423.
Mousapour et al., Efficacy and Safety of Acetylcysteine for the Prevention of Liver Injury in Covid-19 Intensive Care Unit Patients Under Treatment with Remdesivir: A Double-Blind, Placebo-Controlled Randomized Clinical Trial: Prevention of liver injury in severe Covid-19 pneumonia, Gastroenterology and Hepatology from Bed to Bench, doi:10.22037/ghfbb.v15i3.2565.
Rahimi et al., Efficacy of N-acetyl Cysteine in Severe COVID-19 Patients: A Randomized Controlled Phase III Clinical Trial, Jundishapur Journal of Natural Pharmaceutical Products, doi:10.5812/jjnpp-129817.
Çavuş et al., Kritik COVID-19 hastalarında kullanılan N-asetilsisteinin(NAC) klinik bulgulara, inflamatuar parametrelere böbrek fonksiyonlarına olan etkileri, Aksaray Üniversitesi Tıp Bilimleri Dergisi, 3:2, dergipark.org.tr/en/pub/asujms/issue/73171/1062868.
Panahi et al., Evaluation the efficacy and safety of N‐acetylcysteine inhalation spray in controlling the symptoms of patients with COVID‐19: An open‐label randomized controlled clinical trial, Journal of Medical Virology, doi:10.1002/jmv.28393.
Gamarra-Morales et al., Response to Intravenous N-Acetylcysteine Supplementation in Critically Ill Patients with COVID-19, Nutrients, doi:10.3390/nu15092235.
Afaghi et al., N-acetylcysteine as adjuvant therapy for hospitalized Covid-19 patients: A single-center prospective cohort study, Caspian J Intern Med, doi:10.22088/cjim.14.3.553.
Sherkawy et al., Impact of N-Acetylcysteine on Modulating Inflammation in Patients Hospitalized with Moderate COVID-19 Infections: A Prospective Randomized Trial, Archives of Pharmaceutical Sciences Ain Shams University, doi:10.21608/aps.2023.212265.1122.
Galindo-Andúgar et al., Impact of N-Acetylcysteine in the mortality of patients hospitalized with COVID-19: a retrospective cohort study, Revista Clínica Española, doi:10.1016/j.rceng.2023.07.006.
Atefi et al., Evaluation of the efficacy and safety of oral N‐acetylcysteine in patients with COVID‐19 receiving the routine antiviral and hydroxychloroquine protocol: A randomized controlled clinical trial, Immunity, Inflammation and Disease, doi:10.1002/iid3.1083.
Huh (C) et al., Association of previous medications with the risk of COVID-19: a nationwide claims-based study from South Korea, medRxiv, doi:10.1101/2020.05.04.20089904.
Lenze et al., Fluvoxamine vs Placebo and Clinical Deterioration in Outpatients With Symptomatic COVID-19: A Randomized Clinical Trial, JAMA, doi:10.1001/jama.2020.22760.
Seftel et al., Prospective cohort of fluvoxamine for early treatment of COVID-19, Open Forum Infectious Diseases, doi:10.1093/ofid/ofab050.
Reiersen et al., The STOP COVID 2 study: Fluvoxamine vs placebo for outpatients with symptomatic COVID-19, a fully-remote randomized controlled trial, Open Forurm Infectious Diseases, doi:10.1093/ofid/ofad419.
Seo et al., Fluvoxamine Treatment of Patients with Symptomatic COVID-19 in a Community Treatment Center: A Preliminary Result of Randomized Controlled Trial, Infection & Chemotherapy, doi:10.3947/ic.2021.0142.
Bramante (G) et al., Randomized Trial of Metformin, Ivermectin, and Fluvoxamine for Covid-19, NEJM, doi:10.1056/NEJMoa2201662.
Pineda et al., Impact of fluvoxamine on outpatient treatment of COVID-19 in Honduras in a prospective observational real-world study, Frontiers in Pharmacology, doi:10.3389/fphar.2022.1054644.
Farahani et al., Effect of fluvoxamine on preventing neuropsychiatric symptoms of post COVID syndrome in mild to moderate patients, a randomized placebo-controlled double-blind clinical trial, BMC Infectious Diseases, doi:10.1186/s12879-023-08172-5.
Reis (D) et al., Oral Fluvoxamine With Inhaled Budesonide for Treatment of Early-Onset COVID-19, Annals of Internal Medicine, doi:10.7326/M22-3305.
Siripongboonsitti (B) et al., Efficacy of Combination Therapy of Fluvoxamine and Favipiravir versus Favipiravir Monotherapy to Prevent Severe COVID-19 among Mild to Moderate COVID-19 Patients: Open-label Randomized Controlled Trial (EFFaCo Study), International Journal of Infectious Diseases, doi:10.1016/j.ijid.2023.06.018.
Tsiakalos et al., Early Fluvoxamine Reduces the Risk for Clinical Deterioration in Symptomatic Outpatients with COVID-19: A Real-World, Retrospective, before–after Analysis, Microorganisms, doi:10.3390/microorganisms11082073.
Siripongboonsitti (C) et al., The Real-World Effectiveness of Fluvoxamine Therapy in Mild to Moderate COVID-19 Patients; a Historical Cohort Study (Fluvoxa Trial), Journal of Infection and Public Health, doi:10.1016/j.jiph.2023.10.010.
Wannigama et al., Early treatment with fluvoxamine, bromhexine, cyproheptadine, and niclosamide to prevent clinical deterioration in patients with symptomatic COVID-19: a randomized clinical trial, eClinicalMedicine, 10.1016/j.eclinm.2024.102517, www.thelancet.com/journals/eclinm/article/PIIS2589-5370(24)00096-8/fulltext.
Reis (E) et al., Effect of early treatment with fluvoxamine on risk of emergency care and hospitalisation among patients with COVID-19: the TOGETHER randomised, platform clinical trial, The Lancet Global Health, doi:10.1016/S2214-109X(21)00448-4.
Calusic et al., Safety and efficacy of fluvoxamine in COVID-19 ICU patients: an open label, prospective cohort trial with matched controls, British Journal of Clinical Pharmacology, doi:10.1111/bcp.15126.
Kirenga (B) et al., Association of fluvoxamine with mortality and symptom resolution among inpatients with COVID-19 in Uganda: a prospective interventional open-label cohort study, Molecular Psychiatry, doi:10.1038/s41380-023-02004-3.
Stewart (B) et al., Higher-Dose Fluvoxamine and Time to Sustained Recovery in Outpatients With COVID-19, JAMA, doi:10.1001/jama.2023.23363.
Oskotsky et al., Mortality Risk Among Patients With COVID-19 Prescribed Selective Serotonin Reuptake Inhibitor Antidepressants, JAMA Network Open, doi:10.1001/jamanetworkopen.2021.33090.
Fritz et al., Association between antidepressant use and ED or hospital visits in outpatients with SARS-CoV-2, Translational Psychiatry, doi:10.1038/s41398-022-02109-3.
Diaz (B) et al., Protective Effect of Fluvoxamine for COVID-19 in Obsessive-Compulsive Disorder, The Primary Care Companion For CNS Disorders, doi:10.4088/PCC.22br03337.
Trkulja et al., Outpatients prescribed with fluvoxamine around the time of COVID-19 diagnosis are not at a reduced risk of subsequent hospitalization and death compared to their non-prescribed peers: population-based matched cohort study, European Journal of Clinical Pharmacology, doi:10.1007/s00228-023-03479-3.
Visos-Varela et al., Repurposing selective serotonin reuptake inhibitors for severity of COVID-19: A population-based study, European Neuropsychopharmacology, doi:10.1016/j.euroneuro.2023.03.011.
Ashraf (B) et al., Honey and Nigella sativa against COVID-19 in Pakistan (HNS-COVID-PK): A multi-center placebo-controlled randomized clinical trial, Phytotherapy Research, doi:10.1002/ptr.7640.
Al-Haidari et al., Clinical Trial of Black Seeds Against COVID – 19 in Kirkuk City / Iraq, Indian Journal of Forensic Medicine & Toxicology, 15:3 (preprint 1/2021), www.researchgate.net/publication/352134969_Clinical_Trial_of_Black_Seeds_Against_COVID_-19_in_Kirkuk_City_Iraq.
Aldwihi (B) et al., Patients’ Behavior Regarding Dietary or Herbal Supplements before and during COVID-19 in Saudi Arabia, International Journal of Environmental Research and Public Health, doi:10.3390/ijerph18105086.
Koshak et al., Nigella sativa for the treatment of COVID-19: An open-label randomized controlled clinical trial, Complementary Therapies in Medicine, doi:10.1016/j.ctim.2021.102769.
Bencheqroun et al., A Randomized, Double-Blind, Placebo-Controlled, Multicenter Study to Evaluate the Safety and Efficacy of ThymoQuinone Formula (TQF) for Treating Outpatient SARS-CoV-2, Pathogens, doi:10.3390/pathogens11050551.
Said (B) et al., The effect of Nigella sativa and vitamin D3 supplementation on the clinical outcome in COVID-19 patients: A randomized controlled clinical trial, Frontiers in Pharmacology, doi:10.3389/fphar.2022.1011522.
Idris et al., Nigella sativa oil (NSO) as an adjuvant in the management of mild COVID-19 infection in Kaduna state, The Nigerian Health Journal, doi:10.60787/tnhj-712.
Karimi et al., Efficacy of Persian medicine herbal formulations (capsules and decoction) compared to standard care in patients with COVID-19, a multicenter open-labeled, randomized, controlled clinical trial, Phytotherapy Research, doi:10.1002/ptr.7277.
Setayesh et al., Efficacy of a Persian herbal medicine compound on coronavirus disease 2019 (COVID-19): a randomized clinical trial, Integrative Medicine Research, doi:10.1016/j.imr.2022.100869.
Faruq et al., Role of Nigella Sativa (black cumin seeds)as an adjunct therapy in treating severe and critical COVID-19 infection compared to those with standard therapy : An open label randomized clinical trial, Bangladesh Critical Care Journal, doi:10.3329/bccj.v11i2.69187.
Al-Haidari (B) et al., Preventive Value of Black Seed in People at Risk of Infection with COVID-19, Pakistan Journal of Medical and Health Sciences, 15:1, pjmhsonline.com/2021/jan/384.pdf.
Shehab (B) et al., Immune-boosting effect of natural remedies and supplements on progress of, and recovery from COVID-19 infection, Tropical Journal of Pharmaceutical Research, doi:10.4314/tjpr.v21i2.13.
Chandra et al., Efficacy of polyherbal formulations for prevention of COVID-19 infection in high-risk subjects: A randomized open-label controlled clinical trial, Phytotherapy Research, doi:10.1002/ptr.7531.
Daneshfard et al., Effect of Sinamaz nasal drop on asymptomatic family members of COVID 19 patients: An open-label randomized controlled trial, Phytotherapy Research, doi:10.1002/ptr.7915.
Brennan et al., Oral famotidine versus placebo in non-hospitalised patients with COVID-19: a randomised, double-blind, data-intense, phase 2 clinical trial, Gut, doi:10.1136/gutjnl-2022-326952.
Shoaibi (B) et al., Comparative Effectiveness of Famotidine in Hospitalized COVID-19 Patients, American Journal of Gastroenterology, doi:10.14309/ajg.0000000000001153.
Zhou (D) et al., Proton pump inhibitor or famotidine use and severe COVID-19 disease: a propensity score-matched territory-wide study, Gut, doi:10.1136/gutjnl-2020-323668.
Yeramaneni et al., Famotidine Use Is Not Associated With 30-day Mortality: A Coarsened Exact Match Study in 7158 Hospitalized Patients With Coronavirus Disease 2019 From a Large Healthcare System, Gastroenterology, doi:10.1053/j.gastro.2020.10.011.
Mura (B) et al., Real-world evidence for improved outcomes with histamine antagonists and aspirin in 22,560 COVID-19 patients, Signal Transduction and Targeted Therapy, doi:10.1038/s41392-021-00689-y.
Samimagham et al., The Efficacy of Famotidine in improvement of outcomes in Hospitalized COVID-19 Patients: A phase III randomised clinical trial, Research Square, doi:10.21203/rs.3.rs-462937/v1.
Elhadi et al., Epidemiology, outcomes, and utilization of intensive care unit resources for critically ill COVID-19 patients in Libya: A prospective multi-center cohort study, PLOS ONE, doi:10.1371/journal.pone.0251085.
Kuno et al., The association between famotidine and in-hospital mortality of patients with COVID-19, Journal of Medical Virology, doi:10.1002/jmv.27375.
Stolow et al., A Retrospective Review: Famotidine Use Is Not Associated With Improved Outcomes in Hospitalized Patients With COVID-19, American Journal of Gastroenterology, doi:10.14309/01.ajg.0000778736.01714.cd.
Wagner et al., A retrospective analysis of clinical outcomes between hospitalized patients with COVID‐19 who received famotidine or pantoprazole, JGH Open, doi:10.1002/jgh3.12905.
Pahwani et al., Efficacy of Oral Famotidine in Patients Hospitalized With Severe Acute Respiratory Syndrome Coronavirus 2, Cureus, doi:10.7759/cureus.22404.
Siraj et al., Efficacy of Various Treatment Modalities on Patient-related Outcome in Hospitalized COVID-19 Patients – A Retrospective Study, Indian Journal of Clinical Practice, 32:9, ijcp.in/Admin/CMS/PDF/6.%20OriginalResearch_IJCP_Feb2022.pdf.
Zangeneh et al., Survival analysis based on body mass index in patients with Covid-19 admitted to the intensive care unit of Amir Al-Momenin Hospital in Arak – 2021, Obesity Medicine, doi:10.1016/j.obmed.2022.100420.
Chowdhury (B) et al., Role of H2 receptor blocker famotidine over the clinical recovery of COVID-19 patients: A randomized controlled trial, World Journal of Clinical Cases, doi:10.12998/wjcc.v10.i23.8170.
Özden et al., Effects of Famotidine on COVID-19 Patients in Intensive Care Unit: A Retrospective Clinical Trial, Boğazi̇çi̇ Tip Dergi̇si̇, doi:10.14744/bmj.2023.77044.
Freedberg et al., Famotidine Use Is Associated With Improved Clinical Outcomes in Hospitalized COVID-19 Patients: A Propensity Score Matched Retrospective Cohort Study, Gastroenterology, doi:10.1053/j.gastro.2020.05.053.
Mather et al., Impact of Famotidine Use on Clinical Outcomes of Hospitalized Patients With COVID-19, American Journal of Gastroenterology, doi:10.14309/ajg.0000000000000832.
Balouch et al., Role of Famotidine and Other Acid Reflux Medications for SARS-CoV-2: A Pilot Study, Journal of Voice, doi:10.1016/j.jvoice.2021.01.007.
Cheung et al., Association Between Famotidine Use and COVID-19 Severity in Hong Kong: A Territory-wide Study, Gastroenterology, doi:10.1053/j.gastro.2020.05.098.
Fung (B) et al., Effect of common maintenance drugs on the risk and severity of COVID-19 in elderly patients, PLoS ONE, doi:10.1371/journal.pone.0266922.
Razjouyan et al., Smoking Status and Factors associated with COVID-19 In-Hospital Mortality among US Veterans, Nicotine & Tobacco Research, doi:10.1093/ntr/ntab223.
Wallace (B) et al., Association of the patterns of use of medications with mortality of COVID-19 infection: a hospital-based observational study, BMJ Open, doi:10.1136/bmjopen-2021-050051.
MacFadden (D) et al., Screening Large Population Health Databases for Potential COVID-19 Therapeutics: A Pharmacopeia-Wide Association Study (PWAS) of Commonly Prescribed Medications, Open Forum Infectious Diseases, doi:10.1093/ofid/ofac156.
Loucera (D) et al., Real-world evidence with a retrospective cohort of 15,968 COVID-19 hospitalized patients suggests 21 new effective treatments, Virology Journal, doi:10.1186/s12985-023-02195-9.
Kim (D) et al., Histamine-2 Receptor Antagonists and Proton Pump Inhibitors Are Associated With Reduced Risk of SARS-CoV-2 Infection Without Comorbidities Including Diabetes, Hypertension, and Dyslipidemia: A Propensity Score-Matched Nationwide Cohort Study, Journal of Korean Medical Science, doi:10.3346/jkms.2023.38.e99.
Kwon (B) et al., Effectiveness of famotidine on the risk of poor prognosis in patients with COVID-19: A nationwide cohort study in Korea, Heliyon, doi:10.1016/j.heliyon.2023.e16171.
Di Pierro (D) et al., Possible Therapeutic Effects of Adjuvant Quercetin Supplementation Against Early-Stage COVID-19 Infection: A Prospective, Randomized, Controlled, and Open-Label Study, International Journal of General Medicine, doi:10.2147/IJGM.S318720.
Khan (C) et al., Oral Co-Supplementation of Curcumin, Quercetin, and Vitamin D3 as an Adjuvant Therapy for Mild to Moderate Symptoms of COVID-19—Results From a Pilot Open-Label, Randomized Controlled Trial, Frontiers in Pharmacology, doi:10.3389/fphar.2022.898062.
Di Pierro (E) et al., Quercetin as a possible complementary agent for early-stage COVID-19: Concluding results of a randomized clinical trial, Frontiers in Pharmacology, doi:10.3389/fphar.2022.1096853.
Din Ujjan (B) et al., The possible therapeutic role of curcumin and quercetin in the early-stage of COVID-19—Results from a pragmatic randomized clinical trial, Frontiers in Nutrition, doi:10.3389/fnut.2022.1023997.
Onal et al., Treatment of COVID-19 patients with quercetin: a prospective, single center, randomized, controlled trial, Turk. J. Biol., 45:518-529 (preprint 1/19/2021), journals.tubitak.gov.tr/biology/vol45/iss7/13/.
Zupanets et al., Quercetin effectiveness in patients with COVID-19 associated pneumonia, Zaporozhye Med. J., doi:10.14739/2310-1210.2021.5.231714.
Shohan et al., The therapeutic efficacy of quercetin in combination with antiviral drugs in hospitalized COVID-19 patients: A randomized controlled trial, European Journal of Pharmacology, doi:10.1016/j.ejphar.2021.1746158.
Gérain (B) et al., NASAFYTOL® supplementation in adults hospitalized with COVID-19 infection: results from an exploratory open-label randomized controlled trial, Frontiers in Nutrition, doi:10.3389/fnut.2023.1137407.
Tylishchak et al., Effectiveness of the quercetin use in patients with COVID-19 with concomitant type 2 diabetes mellitus, Wiadomości Lekarskie, doi:10.36740/WLek/191875.
Arslan et al., Synergistic Effect of Quercetin and Vitamin C Against COVID-19: Is a Possible Guard for Front Liners, SSRN, doi:10.2139/ssrn.3682517.
Margolin et al., 20-Week Study of Clinical Outcomes of Over-the-Counter COVID-19 Prophylaxis and Treatment, Journal of Evidence-Based Integrative Medicine, doi:10.1177/2515690X211026193.
Rondanelli et al., Promising Effects of 3-Month Period of Quercetin Phytosome® Supplementation in the Prevention of Symptomatic COVID-19 Disease in Healthcare Workers: A Pilot Study, Life, doi:10.3390/life12010066.
González-Paz et al., Biophysical Analysis of Potential Inhibitors of SARS-CoV-2 Cell Recognition and Their Effect on Viral Dynamics in Different Cell Types: A Computational Prediction from In Vitro Experimental Data, ACS Omega, doi:10.1021/acsomega.3c06968.
Alkafaas et al., A study on the effect of natural products against the transmission of B.1.1.529 Omicron, Virology Journal, doi:10.1186/s12985-023-02160-6.
Guimarães Silva et al., Are Non-Structural Proteins From SARS-CoV-2 the Target of Hydroxychloroquine? An in Silico Study, ACTA MEDICA IRANICA, doi:10.18502/acta.v61i2.12533.
Nguyen et al., The Potential of Ameliorating COVID-19 and Sequelae From Andrographis paniculata via Bioinformatics, Bioinformatics and Biology Insights, doi:10.1177/11779322221149622.
Yadav (C) et al., Repurposing the Combination Drug of Favipiravir, Hydroxychloroquine and Oseltamivir as a Potential Inhibitor Against SARS-CoV-2: A Computational Study, Research Square, doi:10.21203/rs.3.rs-628277/v1.
Hussein (B) et al., Molecular Docking Identification for the efficacy of Some Zinc Complexes with Chloroquine and Hydroxychloroquine against Main Protease of COVID-19, Journal of Molecular Structure, doi:10.1016/j.molstruc.2021.129979.
Baildya et al., Inhibitory capacity of Chloroquine against SARS-COV-2 by effective binding with Angiotensin converting enzyme-2 receptor: An insight from molecular docking and MD-simulation studies, Journal of Molecular Structure, doi:10.1016/j.molstruc.2021.129891.
Noureddine et al., Quantum chemical studies on molecular structure, AIM, ELF, RDG and antiviral activities of hybrid hydroxychloroquine in the treatment of COVID-19: molecular docking and DFT calculations, Journal of King Saud University - Science, doi:10.1016/j.jksus.2020.101334.
Tarek et al., Pharmacokinetic Basis of the Hydroxychloroquine Response in COVID-19: Implications for Therapy and Prevention, European Journal of Drug Metabolism and Pharmacokinetics, doi:10.1007/s13318-020-00640-6.
Rowland Yeo et al., Impact of Disease on Plasma and Lung Exposure of Chloroquine, Hydroxychloroquine and Azithromycin: Application of PBPK Modeling, Clinical Pharmacology & Therapeutics, doi:10.1002/cpt.1955.
Hitti et al., Hydroxychloroquine attenuates double-stranded RNA-stimulated hyper-phosphorylation of tristetraprolin/ZFP36 and AU-rich mRNA stabilization, Immunology, doi:10.1111/imm.13835.
Yan et al., Super-resolution imaging reveals the mechanism of endosomal acidification inhibitors against SARS-CoV-2 infection, ChemBioChem, doi:10.1002/cbic.202400404.
Mohd Abd Razak et al., In Vitro Anti-SARS-CoV-2 Activities of Curcumin and Selected Phenolic Compounds, Natural Product Communications, doi:10.1177/1934578X231188861.
Alsmadi et al., The In Vitro, In Vivo, and PBPK Evaluation of a Novel Lung-Targeted Cardiac-Safe Hydroxychloroquine Inhalation Aerogel, AAPS PharmSciTech, doi:10.1208/s12249-023-02627-3.
Wen et al., Cholinergic α7 nAChR signaling suppresses SARS-CoV-2 infection and inflammation in lung epithelial cells, Journal of Molecular Cell Biology, doi:10.1093/jmcb/mjad048.
Kamga Kapchoup et al., In vitro effect of hydroxychloroquine on pluripotent stem cells and their cardiomyocytes derivatives, Frontiers in Pharmacology, doi:10.3389/fphar.2023.1128382.
Milan Bonotto et al., Cathepsin inhibitors nitroxoline and its derivatives inhibit SARS-CoV-2 infection, Antiviral Research, doi:10.1016/j.antiviral.2023.105655.
Miao (B) et al., SIM imaging resolves endocytosis of SARS-CoV-2 spike RBD in living cells, Cell Chemical Biology, doi:10.1016/j.chembiol.2023.02.001.
Yuan (B) et al., Hydroxychloroquine blocks SARS-CoV-2 entry into the endocytic pathway in mammalian cell culture, Communications Biology, doi:10.1038/s42003-022-03841-8.
Faísca et al., Enhanced In Vitro Antiviral Activity of Hydroxychloroquine Ionic Liquids against SARS-CoV-2, Pharmaceutics, doi:10.3390/pharmaceutics14040877.
Delandre et al., Antiviral Activity of Repurposing Ivermectin against a Panel of 30 Clinical SARS-CoV-2 Strains Belonging to 14 Variants, Pharmaceuticals, doi:10.3390/ph15040445.
Purwati (B) et al., An in vitro study of dual drug combinations of anti-viral agents, antibiotics, and/or hydroxychloroquine against the SARS-CoV-2 virus isolated from hospitalized patients in Surabaya, Indonesia, PLOS One, doi:10.1371/journal.pone.0252302.
Zhang (E) et al., SARS-CoV-2 spike protein dictates syncytium-mediated lymphocyte elimination, Cell Death & Differentiation, doi:10.1038/s41418-021-00782-3.
Dang et al., Structural basis of anti-SARS-CoV-2 activity of hydroxychloroquine: specific binding to NTD/CTD and disruption of LLPS of N protein, bioRxiv, doi:10.1101/2021.03.16.435741.
Shang et al., Inhibitors of endosomal acidification suppress SARS-CoV-2 replication and relieve viral pneumonia in hACE2 transgenic mice, Virology Journal, doi:10.1186/s12985-021-01515-1.
Wang (E) et al., Chloroquine and hydroxychloroquine as ACE2 blockers to inhibit viropexis of 2019-nCoV Spike pseudotyped virus, Phytomedicine, doi:10.1016/j.phymed.2020.153333.
Sheaff, R., A New Model of SARS-CoV-2 Infection Based on (Hydroxy)Chloroquine Activity, bioRxiv, doi:10.1101/2020.08.02.232892.
Ou et al., Hydroxychloroquine-mediated inhibition of SARS-CoV-2 entry is attenuated by TMPRSS2, PLOS Pathogens, doi:10.1371/journal.ppat.1009212.
Andreani et al., In vitro testing of combined hydroxychloroquine and azithromycin on SARS-CoV-2 shows synergistic effect, Microbial Pathogenesis, doi:10.1016/j.micpath.2020.104228.
Clementi et al., Combined Prophylactic and Therapeutic Use Maximizes Hydroxychloroquine Anti-SARS-CoV-2 Effects in vitro, Front. Microbiol., 10 July 2020, doi:10.3389/fmicb.2020.01704.
Liu (D) et al., Hydroxychloroquine, a less toxic derivative of chloroquine, is effective in inhibiting SARS-CoV-2 infection in vitro, Cell Discovery 6, 16 (2020), doi:10.1038/s41421-020-0156-0.
Yao et al., In Vitro Antiviral Activity and Projection of Optimized Dosing Design of Hydroxychloroquine for the Treatment of Severe Acute Respiratory Syndrome Coronavirus 2 (SARS-CoV-2), Clin. Infect. Dis., 2020 Mar 9, doi:10.1093/cid/ciaa237.
Wang (F) et al., Remdesivir and chloroquine effectively inhibit the recently emerged novel coronavirus (2019-nCoV) in vitro, Cell Res. 30, 269–271, doi:10.1038/s41422-020-0282-0.
Shu-Han Lin et al., Inhalable Chitosan-Based Hydrogel as a Mucosal Adjuvant for Hydroxychloroquine in the Treatment for SARS-CoV-2 Infection in a Hamster Model, Journal of Microbiology, Immunology and Infection, doi:10.1016/j.jmii.2023.08.001.
Kreutzberger et al., SARS-CoV-2 requires acidic pH to infect cells, Proceedings of the National Academy of Sciences, doi:10.1073/pnas.2209514119.
Al-Bari, M., Targeting endosomal acidification by chloroquine analogs as a promising strategy for the treatment of emerging viral diseases, Pharmacology Research & Perspectives, doi:10.1002/prp2.293.
Ruiz et al., Hydroxychloroquine lung pharmacokinetics in critically ill patients infected with COVID-19, International Journal of Antimicrobial Agents, doi:10.1016/j.ijantimicag.2020.106247.
Chaudhary et al., Impact of prophylactic hydroxychloroquine on ultrastructural impairment and cellular SARS-CoV-2 infection in different cells of bronchoalveolar lavage fluids of COVID-19 patients, Scientific Reports, doi:10.1038/s41598-023-39941-6.
Landsteiner de Sampaio Amêndola et al., COVID-19 Infection in Rheumatic Patients on Chronic Antimalarial Drugs: A Systematic Review and Meta-Analysis, Journal of Clinical Medicine, doi:10.3390/jcm11226865.
García-Albéniz et al., Systematic review and meta-analysis of randomized trials of hydroxychloroquine for the prevention of COVID-19, European Journal of Epidemiology, doi:10.1007/s10654-022-00891-4.
Ladapo et al., Randomized Controlled Trials of Early Ambulatory Hydroxychloroquine in the Prevention of COVID-19 Infection, Hospitalization, and Death: Meta-Analysis, medRxiv, doi:10.1101/2020.09.30.20204693.
Prodromos et al., Hydroxychloroquine is effective, and consistently so used early, for Covid-19: A systematic review, New Microbes and New Infections, doi:10.1016/j.nmni.2020.100776.
Risch, H., Early Outpatient Treatment of Symptomatic, High-Risk Covid-19 Patients that Should be Ramped-Up Immediately as Key to the Pandemic Crisis, American Journal of Epidemiology, kwaa093, 27 May 2020, doi:10.1093/aje/kwaa093.
Risch (B), H., Response to: “Early Outpatient Treatment of Symptomatic, High-Risk Covid-19 Patients” and “Re: Early Outpatient Treatment of Symptomatic, High-Risk Covid-19 Patients that Should be Ramped-Up Immediately as Key to the Pandemic Crisis”, American Journal of Epidemiology, July 20, 2020, doi:10.1093/aje/kwaa152.
Stricker et al., Hydroxychloroquine Pre-Exposure Prophylaxis for COVID-19 in Healthcare Workers from India: A Meta-Analysis, Journal of Infection and Public Health, doi:10.1016/j.jiph.2021.08.001.
Han (B) et al., The efficacy and safety of hydroxychloroquine for COVID-19 prophylaxis and clinical assessment: an updated meta-analysis of randomized trials, Journal of Thoracic Disease, doi:10.21037/jtd-23-1043.
Michelucci et al., SARS-CoV-2 ORF3a accessory protein is a water-permeable channel that induces lysosome swelling, Communications Biology, doi:10.1038/s42003-024-07442-5.
Ghosh et al., β-Coronaviruses Use Lysosomes for Egress Instead of the Biosynthetic Secretory Pathway, Cell, doi:10.1016/j.cell.2020.10.039.
Axfors et al., Mortality outcomes with hydroxychloroquine and chloroquine in COVID-19 from an international collaborative meta-analysis of randomized trials, Nature, doi:10.1038/s41467-021-22446-z.
Kim (E) et al., Comparison of Lopinavir/Ritonavir or Hydroxychloroquine in Patients With Mild Coronavirus Disease (COVID-19), NCT04307693, clinicaltrials.gov/study/NCT04438837.
Butler et al., PRINCIPLE: A clinical trial evaluating treatments for suspected and confirmed COVID-19 for recovery at home, PRINCIPLE, www.isrctn.com/ISRCTN86534580.
Sarwar et al., PRophylaxis of Exposed COVID-19 Individuals With Mild Symptoms Using choloroquinE Compounds (PRECISE), NCT04351191, clinicaltrials.gov/study/NCT04351191.
Sow et al., Phytomedicines Versus Hydroxychloroquine as an Add on Therapy to Azythromycin in Asymptomatic Covid-19 Patients (PHYTCOVID-19), NCT04501965, clinicaltrials.gov/study/NCT04501965.
Okasha et al., Hydroxychloroquine and Nitazoxanide Combination Therapy for COVID-19, NCT04361318, clinicaltrials.gov/study/NCT04361318.
Gül et al., Clinical Trial For Early SARS-CoV-2 (COVID-19) Treatment, NCT04981379, clinicaltrials.gov/study/NCT04981379.
Kara et al., Efficacy and Safety of Hydroxychloroquine and Favipiravir in the Treatment of Mild to Moderate COVID-19, NCT04411433, clinicaltrials.gov/study/NCT04411433.
Abayomi et al., A multi-centre, randomized, double-blind, placebo-controlled clinical trial of the efficacy and safety of chloroquine phosphate, hydroxychloroquine sulphate and lopinavir/ritonavir for the treatment of COVID-19 in Lagos State: study protocol for a randomized controlled trial, Trials, doi:10.1186/s13063-021-05675-x.
Aston et al., Hydroxychloroquine vs. Azithromycin for Outpatients in Utah With COVID-19 (HyAzOUT), NCT04334382, clinicaltrials.gov/study/NCT04334382.
Pineda (B) et al., Prevention and Treatment With Hydroxychloroquine + Azithromycin of Acute Respiratory Syndrome Induced by COVID-19 (AMBUCOV), NCT04954040, clinicaltrials.gov/study/NCT04954040.
Genton et al., #StayHome: Early Hydroxychloroquine to Reduce Secondary Hospitalisation and Household Transmission in COVID-19 (#StayHome), NCT04385264, clinicaltrials.gov/study/NCT04385264.
Farooq et al., Effectiveness of Hydroxychloroquine in Covid-19 Patients (Covid), NCT04328272, clinicaltrials.gov/study/NCT04328272.
Mežnar et al., Use of Bromhexine and Hydroxychloroquine for Treatment of COVID-19 Pneumonia, NCT04355026, clinicaltrials.gov/study/NCT04355026.
El-Sherbiny et al., Development and Validation of "Ready-to-Use" Inhalable Forms of Hydroxychloroquine for Treatment of COVID-19, NCT04477083, clinicaltrials.gov/study/NCT04477083.
WellStar, Hydroxychloroquine Use in Hospitalized Patients With COVID-19: Impact on Progression to Severe or Critical Disease, NCT04429867, clinicaltrials.gov/study/NCT04429867.
Levi et al., Open Label Study to Compare Efficacy, Safety and Tolerability of Hydroxychloroquine Combined With Azithromycin Compared to Hydroxychloroquine Combined With Camostat Mesylate and to "no Treatment" in SARS CoV 2 Virus (COSTA), NCT04355052, clinicaltrials.gov/study/NCT04355052.
Mordmüller et al., Hydroxychloroquine for COVID-19 (COV-HCQ), NCT04342221, clinicaltrials.gov/study/NCT04342221.
Hawari et al., The Potential Use of Nebulized Hydroxychloroquine for the Treatment of COVID-19, NCT05113810, clinicaltrials.gov/study/NCT05113810.
Treluyer et al., Prevention of SARS-CoV-2 in Hospital Workers s Exposed to the Virus (PREP-COVID), PREP-COVID, NCT04344379, clinicaltrials.gov/study/NCT04344379.
Niriella et al., Hydroxychloroquine for post-exposure prophylaxis of COVID-19 among naval personnel in Sri Lanka: study protocol for a randomized, controlled trial, Trials, doi:10.1186/s13063-020-04659-7.
Ajili et al., A Study of Hydroxychloroquine and Zinc in the Prevention of COVID-19 Infection in Military Healthcare Workers (COVID-Milit), NCT04377646, clinicaltrials.gov/study/NCT04377646.
Connor et al., HEalth Care Worker pROphylaxis Against COVID-19: The HERO Trial (HERO), NCT04352946, clinicaltrials.gov/study/NCT04352946.
Pellegrini (B) et al., Effectiveness of Prophylactic Hydroxychloroquine on incidence of COVID-19 infection in Front-line Health and Allied Health Care Workers: The COVID-SHIELD Trial, COVID-SHIELD, ACTRN12620000501943, www.anzctr.org.au/TrialSearch.aspx#&&conditionCode=&dateOfRegistrationFrom=&interventionDescription=&interventionCodeOperator=OR&primarySponsorType=&gender=&distance=&postcode=&pageSize=20&ageGroup=&recruitmentCountryOperator=OR&recruitmentRegion=ðicsReview=&countryOfRecruitment=Australia%7cNew+Zealand®istry=&searchTxt=ACTRN12620000501943.
Burney et al., Hydroxychloroquine Chemoprophylaxis for COVID-19 Infection in High-risk Healthcare Workers, NCT04370015, clinicaltrials.gov/study/NCT04370015.
Morales-Asencio et al., Prevention of COVID19 Infection in Nursing Homes by Chemoprophylaxis With Hydroxychloroquine, NCT04400019, clinicaltrials.gov/study/NCT04400019.
James et al., PROLIFIC ChemoprophylaxisTrial (COVID-19), NCT04352933, clinicaltrials.gov/study/NCT04352933.
Moraes et al., Comparative Study of Hydroxychloroquine and Ivermectin in COVID-19 Prophylaxis, NCT04384458, clinicaltrials.gov/study/NCT04384458.
Chauffe et al., Hydroxychloroquine as Prophylaxis for COVID-19 in Healthcare Workers (HCQPreP), NCT04363450, clinicaltrials.gov/study/NCT04363450.
Granados-Montiel et al., New prophylaxis regimen for SARS-CoV-2 infection in health professionals with low doses of hydroxychloroquine and bromhexine: a randomised, double-blind placebo clinical trial (ELEVATE Trial), BMJ Open, doi:10.1136/bmjopen-2020-045190.
Nanni et al., PROTECT Trial: A cluster-randomized study with hydroxychloroquine versus observational support for prevention or early-phase treatment of Coronavirus disease (COVID-19): A structured summary of a study protocol for a randomized controlled trial, Trials, doi:10.1186/s13063-020-04527-4.
Gagneux-Brunon et al., Acceptability of a COVID-19 pre-exposure prophylaxis trial with hydroxychloroquine in French healthcare workers during the first wave of COVID-19 pandemic, Trials, doi:10.1186/s13063-021-05329-y.
Sarwar (B) et al., Post-Exposure Prophylaxis for Asymptomatic SARS-CoV-2 COVID-19 Patients With choloroquinE Compounds (PEACE), NCT04346667, clinicaltrials.gov/study/NCT04346667.
Abu-Helalah et al., Chemoprevention Clinical Trial of COVID-19: Hydroxychloroquine Post Exposure Prophylaxis (APCC-19), NCT04597775, clinicaltrials.gov/study/NCT04597775.
Borrie et al., COVID-19 PEP- High-risk Individuals in Long-term and Specialized Care - Canada, NCT04397328, clinicaltrials.gov/study/NCT04397328.
González et al., Hydroxychloroquine efficacy and safety in preventing SARS-CoV-2 infection and COVID-19 disease severity during pregnancy (COVID-Preg): a structured summary of a study protocol for a randomised placebo controlled trial, Trials, doi:10.1186/s13063-020-04557-y.
Al Ansari et al., Post Exposure Prophylaxis in Healthcare Workers Exposed to COVID-19 Patients (HCQ-COVID19), NCT04437693, clinicaltrials.gov/study/NCT04437693.
Ghanem-Zoubi et al., Hydroxychloroquine Post-Exposure Prophylaxis for Coronavirus Disease (COVID-19) Among Health-Care Workers, NCT04438837, clinicaltrials.gov/study/NCT04438837.
Million (B) et al., Cardiovascular Safety of Hydroxychloroquine-Azithromycin in 424 COVID-19 Patients, Preprints, doi:10.20944/preprints202303.0325.v1.
Kowatsch et al., Hydroxychloroquine reduces T cells activation recall antigen responses, PLOS ONE, doi:10.1371/journal.pone.0287738.
Tchounga et al., Composition analysis of falsified chloroquine phosphate samples seized during the COVID-19 pandemic, Journal of Pharmaceutical and Biomedical Analysis, doi:10.1016/j.jpba.2020.113761.
Gnegel et al., Identification of Falsified Chloroquine Tablets in Africa at the Time of the COVID-19 Pandemic, The American Journal of Tropical Medicine and Hygiene, doi:10.4269/ajtmh.20-0363.
Gayozo et al., Binding affinities analysis of ivermectin, nucleocapsid and ORF6 proteins of SARS-CoV-2 to human importins α isoforms: A computational approach, Biotecnia, doi:10.18633/biotecnia.v27.2485.
Lefebvre et al., Characterization and Fluctuations of an Ivermectin Binding Site at the Lipid Raft Interface of the N-Terminal Domain (NTD) of the Spike Protein of SARS-CoV-2 Variants, Viruses, doi:10.3390/v16121836.
Haque et al., Exploring potential therapeutic candidates against COVID-19: a molecular docking study, Discover Molecules, doi:10.1007/s44345-024-00005-5.
Bagheri-Far et al., Non-spike protein inhibition of SARS-CoV-2 by natural products through the key mediator protein ORF8, Molecular Biology Research Communications, doi:10.22099/mbrc.2024.50245.2001.
de Oliveira Só et al., In Silico Comparative Analysis of Ivermectin and Nirmatrelvir Inhibitors Interacting with the SARS-CoV-2 Main Protease, Preprints, doi:10.20944/preprints202404.1825.v1.
Agamah et al., Network-based multi-omics-disease-drug associations reveal drug repurposing candidates for COVID-19 disease phases, ScienceOpen, doi:10.58647/DRUGARXIV.PR000010.v1.
Oranu et al., Validation of the binding affinities and stabilities of ivermectin and moxidectin against SARS-CoV-2 receptors using molecular docking and molecular dynamics simulation, GSC Biological and Pharmaceutical Sciences, doi:10.30574/gscbps.2024.26.1.0030.
Zhao et al., Identification of the shared gene signatures between pulmonary fibrosis and pulmonary hypertension using bioinformatics analysis, Frontiers in Immunology, doi:10.3389/fimmu.2023.1197752.
Vottero et al., Computational Prediction of the Interaction of Ivermectin with Fibrinogen, Molecular Sciences, doi:10.3390/ijms241411449.
Chellasamy et al., Docking and molecular dynamics studies of human ezrin protein with a modelled SARS-CoV-2 endodomain and their interaction with potential invasion inhibitors, Journal of King Saud University - Science, doi:10.1016/j.jksus.2022.102277.
Umar et al., Inhibitory potentials of ivermectin, nafamostat, and camostat on spike protein and some nonstructural proteins of SARS-CoV-2: Virtual screening approach, Jurnal Teknologi Laboratorium, doi:10.29238/teknolabjournal.v11i1.344.
Alvarado et al., Interaction of the New Inhibitor Paxlovid (PF-07321332) and Ivermectin With the Monomer of the Main Protease SARS-CoV-2: A Volumetric Study Based on Molecular Dynamics, Elastic Networks, Classical Thermodynamics and SPT, Computational Biology and Chemistry, doi:10.1016/j.compbiolchem.2022.107692.
Aminpour et al., In Silico Analysis of the Multi-Targeted Mode of Action of Ivermectin and Related Compounds, Computation, doi:10.3390/computation10040051.
Parvez et al., Insights from a computational analysis of the SARS-CoV-2 Omicron variant: Host–pathogen interaction, pathogenicity, and possible drug therapeutics, Immunity, Inflammation and Disease, doi:10.1002/iid3.639.
Francés-Monerris et al., Microscopic interactions between ivermectin and key human and viral proteins involved in SARS-CoV-2 infection, Physical Chemistry Chemical Physics, doi:10.1039/D1CP02967C.
González-Paz (B) et al., Comparative study of the interaction of ivermectin with proteins of interest associated with SARS-CoV-2: A computational and biophysical approach, Biophysical Chemistry, doi:10.1016/j.bpc.2021.106677.
González-Paz (C) et al., Structural Deformability Induced in Proteins of Potential Interest Associated with COVID-19 by binding of Homologues present in Ivermectin: Comparative Study Based in Elastic Networks Models, Journal of Molecular Liquids, doi:10.1016/j.molliq.2021.117284.
Rana et al., A Computational Study of Ivermectin and Doxycycline Combination Drug Against SARS-CoV-2 Infection, Research Square, doi:10.21203/rs.3.rs-755838/v1.
Muthusamy et al., Virtual Screening Reveals Potential Anti-Parasitic Drugs Inhibiting the Receptor Binding Domain of SARS-CoV-2 Spike protein, Journal of Virology & Antiviral Research, www.scitechnol.com/abstract/virtual-screening-reveals-potential-antiparasitic-drugs-inhibiting-the-receptor-binding-domain-of-sarscov2-spike-protein-16398.html.
Qureshi et al., Mechanistic insights into the inhibitory activity of FDA approved ivermectin against SARS-CoV-2: old drug with new implications, Journal of Biomolecular Structure and Dynamics, doi:10.1080/07391102.2021.1906750.
Schöning et al., Highly-transmissible Variants of SARS-CoV-2 May Be More Susceptible to Drug Therapy Than Wild Type Strains, Research Square, doi:10.21203/rs.3.rs-379291/v1.
Bello et al., Elucidation of the inhibitory activity of ivermectin with host nuclear importin α and several SARS-CoV-2 targets, Journal of Biomolecular Structure and Dynamics, doi:10.1080/07391102.2021.1911857.
Udofia et al., In silico studies of selected multi-drug targeting against 3CLpro and nsp12 RNA-dependent RNA-polymerase proteins of SARS-CoV-2 and SARS-CoV, Network Modeling Analysis in Health Informatics and Bioinformatics, doi:10.1007/s13721-021-00299-2.
Choudhury et al., Exploring the binding efficacy of ivermectin against the key proteins of SARS-CoV-2 pathogenesis: an in silico approach, Future Medicine, doi:10.2217/fvl-2020-0342.
Kern et al., Modeling of SARS-CoV-2 Treatment Effects for Informed Drug Repurposing, Frontiers in Pharmacology, doi:10.3389/fphar.2021.625678.
Saha et al., The Binding mechanism of ivermectin and levosalbutamol with spike protein of SARS-CoV-2, Structural Chemistry, doi:10.1007/s11224-021-01776-0.
Eweas et al., Molecular Docking Reveals Ivermectin and Remdesivir as Potential Repurposed Drugs Against SARS-CoV-2, Frontiers in Microbiology, doi:10.3389/fmicb.2020.592908.
Parvez (B) et al., Prediction of potential inhibitors for RNA-dependent RNA polymerase of SARS-CoV-2 using comprehensive drug repurposing and molecular docking approach, International Journal of Biological Macromolecules, doi:10.1016/j.ijbiomac.2020.09.098.
Francés-Monerris (B) et al., Has Ivermectin Virus-Directed Effects against SARS-CoV-2? Rationalizing the Action of a Potential Multitarget Antiviral Agent, ChemRxiv, doi:10.26434/chemrxiv.12782258.v1.
Kalhor et al., Repurposing of the approved small molecule drugs in order to inhibit SARS-CoV-2 S protein and human ACE2 interaction through virtual screening approaches, Journal of Biomolecular Structure and Dynamics, doi:10.1080/07391102.2020.1824816.
Swargiary, A., Ivermectin as a promising RNA-dependent RNA polymerase inhibitor and a therapeutic drug against SARS-CoV2: Evidence from in silico studies, Research Square, doi:10.21203/rs.3.rs-73308/v1.
Maurya, D., A Combination of Ivermectin and Doxycycline Possibly Blocks the Viral Entry and Modulate the Innate Immune Response in COVID-19 Patients, American Chemical Society (ACS), doi:10.26434/chemrxiv.12630539.v1.
Lehrer et al., Ivermectin Docks to the SARS-CoV-2 Spike Receptor-binding Domain Attached to ACE2, In Vivo, 34:5, 3023-3026, doi:10.21873/invivo.12134.
Suravajhala et al., Comparative Docking Studies on Curcumin with COVID-19 Proteins, Preprints, doi:10.20944/preprints202005.0439.v3.
Shahin et al., The selective effect of Ivermectin on different human coronaviruses; in-vitro study, Research Square, doi:10.21203/rs.3.rs-4180797/v1.
Jitobaom et al., Identification of inositol monophosphatase as a broad‐spectrum antiviral target of ivermectin, Journal of Medical Virology, doi:10.1002/jmv.29552.
Fauquet et al., Microfluidic Diffusion Sizing Applied to the Study of Natural Products and Extracts That Modulate the SARS-CoV-2 Spike RBD/ACE2 Interaction, Molecules, doi:10.3390/molecules28248072.
García-Aguilar et al., In Vitro Analysis of SARS-CoV-2 Spike Protein and Ivermectin Interaction, International Journal of Molecular Sciences, doi:10.3390/ijms242216392.
Liu (E) et al., SARS-CoV-2 viral genes Nsp6, Nsp8, and M compromise cellular ATP levels to impair survival and function of human pluripotent stem cell-derived cardiomyocytes, Stem Cell Research & Therapy, doi:10.1186/s13287-023-03485-3.
Boschi et al., SARS-CoV-2 Spike Protein Induces Hemagglutination: Implications for COVID-19 Morbidities and Therapeutics and for Vaccine Adverse Effects, bioRxiv, doi:10.1101/2022.11.24.517882.
De Forni et al., Synergistic drug combinations designed to fully suppress SARS-CoV-2 in the lung of COVID-19 patients, PLoS ONE, doi:10.1371/journal.pone.0276751.
Saha (B) et al., Manipulation of Spray-Drying Conditions to Develop an Inhalable Ivermectin Dry Powder, Pharmaceutics, doi:10.3390/pharmaceutics14071432.
Jitobaom (B) et al., Synergistic anti-SARS-CoV-2 activity of repurposed anti-parasitic drug combinations, BMC Pharmacology and Toxicology, doi:10.1186/s40360-022-00580-8.
Croci et al., Liposomal Systems as Nanocarriers for the Antiviral Agent Ivermectin, International Journal of Biomaterials, doi:10.1155/2016/8043983.
Zheng et al., Red blood cell-hitchhiking mediated pulmonary delivery of ivermectin: Effects of nanoparticle properties, International Journal of Pharmaceutics, doi:10.1016/j.ijpharm.2022.121719.
Delandre (B) et al., Antiviral Activity of Repurposing Ivermectin against a Panel of 30 Clinical SARS-CoV-2 Strains Belonging to 14 Variants, Pharmaceuticals, doi:10.3390/ph15040445.
Liu (F) et al., Genome-wide analyses reveal the detrimental impacts of SARS-CoV-2 viral gene Orf9c on human pluripotent stem cell-derived cardiomyocytes, Stem Cell Reports, doi:10.1016/j.stemcr.2022.01.014.
Segatori et al., Effect of Ivermectin and Atorvastatin on Nuclear Localization of Importin Alpha and Drug Target Expression Profiling in Host Cells from Nasopharyngeal Swabs of SARS-CoV-2- Positive Patients, Viruses, doi:10.3390/v13102084.
Jitobaom (C) et al., Favipiravir and Ivermectin Showed in Vitro Synergistic Antiviral Activity against SARS-CoV-2, Research Square, doi:10.21203/rs.3.rs-941811/v1.
Munson et al., Niclosamide and ivermectin modulate caspase-1 activity and proinflammatory cytokine secretion in a monocytic cell line, British Society For Nanomedicine Early Career Researcher Summer Meeting, 2021, web.archive.org/web/20230401070026/https://michealmunson.github.io/COVID.pdf.
Mountain Valley MD, Mountain Valley MD Receives Successful Results From BSL-4 COVID-19 Clearance Trial on Three Variants Tested With Ivectosol™, www.globenewswire.com/en/news-release/2021/05/18/2231755/0/en/Mountain-Valley-MD-Receives-Successful-Results-From-BSL-4-COVID-19-Clearance-Trial-on-Three-Variants-Tested-With-Ivectosol.html.
Yesilbag et al., Ivermectin also inhibits the replication of bovine respiratory viruses (BRSV, BPIV-3, BoHV-1, BCoV and BVDV) in vitro, Virus Research, doi:10.1016/j.virusres.2021.198384.
Mody et al., Identification of 3-chymotrypsin like protease (3CLPro) inhibitors as potential anti-SARS-CoV-2 agents, Communications Biology, doi:10.1038/s42003-020-01577-x.
Jeffreys et al., Remdesivir-ivermectin combination displays synergistic interaction with improved in vitro activity against SARS-CoV-2, International Journal of Antimicrobial Agents, doi:10.1016/j.ijantimicag.2022.106542.
Surnar et al., Clinically Approved Antiviral Drug in an Orally Administrable Nanoparticle for COVID-19, ACS Pharmacol. Transl. Sci., doi:10.1021/acsptsci.0c00179.
Li (H) et al., Quantitative proteomics reveals a broad-spectrum antiviral property of ivermectin, benefiting for COVID-19 treatment, J. Cellular Physiology, doi:10.1002/jcp.30055.
Caly et al., The FDA-approved drug ivermectin inhibits the replication of SARS-CoV-2 in vitro, Antiviral Research, doi:10.1016/j.antiviral.2020.104787.
Zhang (F) et al., Ivermectin inhibits LPS-induced production of inflammatory cytokines and improves LPS-induced survival in mice, Inflammation Research, doi:10.1007/s00011-008-8007-8.
Gao (B) et al., Ivermectin ameliorates acute myocarditis via the inhibition of importin-mediated nuclear translocation of NF-κB/p65, International Immunopharmacology, doi:10.1016/j.intimp.2024.112073.
Abd-Elmawla et al., Suppression of NLRP3 inflammasome by ivermectin ameliorates bleomycin-induced pulmonary fibrosis, Journal of Zhejiang University-SCIENCE B, doi:10.1631/jzus.B2200385.
Uematsu et al., Prophylactic administration of ivermectin attenuates SARS-CoV-2 induced disease in a Syrian Hamster Model, The Journal of Antibiotics, doi:10.1038/s41429-023-00623-0.
Albariqi et al., Pharmacokinetics and Safety of Inhaled Ivermectin in Mice as a Potential COVID-19 Treatment, International Journal of Pharmaceutics, doi:10.1016/j.ijpharm.2022.121688.
Errecalde et al., Safety and Pharmacokinetic Assessments of a Novel Ivermectin Nasal Spray Formulation in a Pig Model, Journal of Pharmaceutical Sciences, doi:10.1016/j.xphs.2021.01.017.
Madrid et al., Safety of oral administration of high doses of ivermectin by means of biocompatible polyelectrolytes formulation, Heliyon, doi:10.1016/j.heliyon.2020.e05820.
Ma (B) et al., Ivermectin contributes to attenuating the severity of acute lung injury in mice, Biomedicine & Pharmacotherapy, doi:10.1016/j.biopha.2022.113706.
de Melo et al., Attenuation of clinical and immunological outcomes during SARS-CoV-2 infection by ivermectin, EMBO Mol. Med., doi:10.15252/emmm.202114122.
Arévalo et al., Ivermectin reduces in vivo coronavirus infection in a mouse experimental model, Scientific Reports, doi:10.1038/s41598-021-86679-0.
Chaccour (B) et al., Nebulized ivermectin for COVID-19 and other respiratory diseases, a proof of concept, dose-ranging study in rats, Scientific Reports, doi:10.1038/s41598-020-74084-y.
Yan (B) et al., Anti-inflammatory effects of ivermectin in mouse model of allergic asthma, Inflammation Research, doi:10.1007/s00011-011-0307-8.
Götz et al., Influenza A viruses escape from MxA restriction at the expense of efficient nuclear vRNP import, Scientific Reports, doi:10.1038/srep23138.
Tay et al., Nuclear localization of dengue virus (DENV) 1–4 non-structural protein 5; protection against all 4 DENV serotypes by the inhibitor Ivermectin, Antiviral Research, doi:10.1016/j.antiviral.2013.06.002.
Wagstaff et al., Ivermectin is a specific inhibitor of importin α/β-mediated nuclear import able to inhibit replication of HIV-1 and dengue virus, Biochemical Journal, doi:10.1042/BJ20120150.
Wagstaff (B) et al., An AlphaScreen®-Based Assay for High-Throughput Screening for Specific Inhibitors of Nuclear Import, SLAS Discovery, doi:10.1177/1087057110390360.
Barrows et al., A Screen of FDA-Approved Drugs for Inhibitors of Zika Virus Infection, Cell Host & Microbe, doi:10.1016/j.chom.2016.07.004.
Yang (B) et al., The broad spectrum antiviral ivermectin targets the host nuclear transport importin α/β1 heterodimer, Antiviral Research, doi:10.1016/j.antiviral.2020.104760.
Mastrangelo et al., Ivermectin is a potent inhibitor of flavivirus replication specifically targeting NS3 helicase activity: new prospects for an old drug, Journal of Antimicrobial Chemotherapy, doi:10.1093/jac/dks147.
Varghese et al., Discovery of berberine, abamectin and ivermectin as antivirals against chikungunya and other alphaviruses, Antiviral Research, doi:10.1016/j.antiviral.2015.12.012.
Bennett et al., Role of a nuclear localization signal on the minor capsid Proteins VP2 and VP3 in BKPyV nuclear entry, Virology, doi:10.1016/j.virol.2014.10.013.
Kosyna et al., The importin α/β-specific inhibitor Ivermectin affects HIF-dependent hypoxia response pathways, Biological Chemistry, doi:10.1515/hsz-2015-0171.
Scheim et al., Sialylated Glycan Bindings from SARS-CoV-2 Spike Protein to Blood and Endothelial Cells Govern the Severe Morbidities of COVID-19, International Journal of Molecular Sciences, doi:10.3390/ijms242317039.
Liu (G) et al., Crosstalk between neutrophil extracellular traps and immune regulation: insights into pathobiology and therapeutic implications of transfusion-related acute lung injury, Frontiers in Immunology, doi:10.3389/fimmu.2023.1324021.
Shouman (B) et al., SARS-CoV-2-associated lymphopenia: possible mechanisms and the role of CD147, Cell Communication and Signaling, doi:10.1186/s12964-024-01718-3.
Scheim (B), D., Ivermectin for COVID-19 Treatment: Clinical Response at Quasi-Threshold Doses Via Hypothesized Alleviation of CD147-Mediated Vascular Occlusion, SSRN, doi:10.2139/ssrn.3636557.
Scheim (C), D., From Cold to Killer: How SARS-CoV-2 Evolved without Hemagglutinin Esterase to Agglutinate and Then Clot Blood Cells, Center for Open Science, doi:10.31219/osf.io/sgdj2.
Behl et al., CD147-spike protein interaction in COVID-19: Get the ball rolling with a novel receptor and therapeutic target, Science of The Total Environment, doi:10.1016/j.scitotenv.2021.152072.
DiNicolantonio et al., Ivermectin may be a clinically useful anti-inflammatory agent for late-stage COVID-19, Open Heart, doi:10.1136/openhrt-2020-001350.
Hazan (B) et al., Treatment with Ivermectin Increases the Population of Bifidobacterium in the Gut, ACG 2023, acg2023posters.eventscribe.net/posterspeakers.asp.
DiNicolantonio (B) et al., Anti-inflammatory activity of ivermectin in late-stage COVID-19 may reflect activation of systemic glycine receptors, Open Heart, doi:10.1136/openhrt-2021-001655.
Descotes, J., Medical Safety of Ivermectin, ImmunoSafe Consultance, web.archive.org/web/20240313025927/https://www.medincell.com/wp-content/uploads/2021/03/Clinical_Safety_of_Ivermectin-March_2021.pdf.
Schwartz (B), E., Does ivermectin have a place in the treatment of mild Covid-19?, New Microbes and New Infections, doi:10.1016/j.nmni.2022.100989.
Bryant et al., Ivermectin for Prevention and Treatment of COVID-19 Infection: A Systematic Review, Meta-analysis, and Trial Sequential Analysis to Inform Clinical Guidelines, American Journal of Therapeutics, doi:10.1097/MJT.0000000000001402.
Hariyanto et al., Ivermectin and outcomes from Covid-19 pneumonia: A systematic review and meta-analysis of randomized clinical trial studies, Reviews In Medical Virology, doi:10.1002/rmv.2265.
Kory et al., Review of the Emerging Evidence Demonstrating the Efficacy of Ivermectin in the Prophylaxis and Treatment of COVID-19, American Journal of Therapeutics, doi:10.1097/MJT.0000000000001377.
Lawrie et al., Ivermectin reduces the risk of death from COVID-19 – a rapid review and meta-analysis in support of the recommendation of the Front Line COVID-19 Critical Care Alliance, Preprint, b3d2650e-e929-4448-a527-4eeb59304c7f.filesusr.com/ugd/593c4f_8cb655bd21b1448ba6cf1f4c59f0d73d.pdf.
Nardelli et al., Crying wolf in time of Corona: the strange case of ivermectin and hydroxychloroquine. Is the fear of failure withholding potential life-saving treatment from clinical use?, Signa Vitae, doi:10.22514/sv.2021.043.
Zein et al., Ivermectin and mortality in patients with COVID-19: A systematic review, meta-analysis, and meta-regression of randomized controlled trials, Diabetes & Metabolic Syndrome: Clinical Research & Reviews, doi:10.1016/j.dsx.2021.102186.
doyourownresearch.substack.com, doyourownresearch.substack.com/p/is-ivermectin-literature-particularly.
Guzzo et al., Safety, Tolerability, and Pharmacokinetics of Escalating High Doses of Ivermectin in Healthy Adult Subjects, J. Clinical Pharmacology, doi:10.1177/009127002237994.
Williams, T., Not All Ivermectin Is Created Equal: Comparing The Quality of 11 Different Ivermectin Sources, Do Your Own Research, doyourownresearch.substack.com/p/not-all-ivermectin-is-created-equal.
Vanhee et al., Substandard and falsified ivermectin tablets obtained for self‐medication during the COVID‐19 pandemic as a source of potential harm, Drug Testing and Analysis, doi:10.1002/dta.3618.
Therapeutic Goods Administration, TGA warns about imports of ivermectin, News Release, December 2021, www.tga.gov.au/news/media-releases/tga-warns-about-imports-ivermectin.
Therapeutic Goods Administration (B), TGA cautions consumers over counterfeit ivermectin, News Release, March 2022, www.tga.gov.au/news/media-releases/tga-cautions-consumers-over-counterfeit-ivermectin.
Huerta León et al., Content and characteristics of ivermectin in master formulations, Revista Cubana de Medicina Militar, 53:4, revmedmilitar.sld.cu/index.php/mil/article/view/45001.
Yemeke et al., Impact of the COVID-19 pandemic on the quality of medical products in Zimbabwe: a qualitative study based on key informant interviews with health system stakeholders, BMJ Open, doi:10.1136/bmjopen-2022-068923.
Tavares et al., Investigation of Interactions Between the Protein MPro and the Vanadium Complex VO(metf)2∙H2O: A Computational Approach for COVID-19 Treatment, Biophysica, doi:10.3390/biophysica5010004.
Hou et al., Metformin is a potential therapeutic for COVID-19/LUAD by regulating glucose metabolism, Scientific Reports, doi:10.1038/s41598-024-63081-0.
Lockwood, T., Coordination chemistry suggests that independently observed benefits of metformin and Zn2+ against COVID-19 are not independent, BioMetals, doi:10.1007/s10534-024-00590-5.
Joshi et al., Severe SARS‐CoV‐2 infection in diabetes was rescued in mice supplemented with metformin and/or αKG, and patients taking metformin, via HIF1α‐IFN axis, Clinical and Translational Medicine, doi:10.1002/ctm2.70275.
Chang et al., SARS-CoV-2 Spike Protein 1 Causes Aggregation of α-Synuclein via Microglia-Induced Inflammation and Production of Mitochondrial ROS: Potential Therapeutic Applications of Metformin, Biomedicines, doi:10.3390/biomedicines12061223.
Yang (C) et al., SARS-CoV-2 infection causes dopaminergic neuron senescence, Cell Stem Cell, doi:10.1016/j.stem.2023.12.012.
Parthasarathy et al., Metformin Suppresses SARS-CoV-2 in Cell Culture, bioRxiv, doi:10.1101/2021.11.18.469078.
Taher (B) et al., Anti‑inflammatory effect of metformin against an experimental model of LPS‑induced cytokine storm, Experimental and Therapeutic Medicine, doi:10.3892/etm.2023.12114.
Wang (G) et al., Effects of metformin on acute respiratory distress syndrome in preclinical studies: a systematic review and meta-analysis, Frontiers in Pharmacology, doi:10.3389/fphar.2023.1215307.
Zhang (G) et al., SARS-CoV-2 ORF3a Protein as a Therapeutic Target against COVID-19 and Long-Term Post-Infection Effects, Pathogens, doi:10.3390/pathogens13010075.
Monsalve et al., NETosis: A key player in autoimmunity, COVID-19, and long COVID, Journal of Translational Autoimmunity, doi:10.1016/j.jtauto.2025.100280.
Petakh et al., Metformin Alters mRNA Expression of FOXP3, RORC, and TBX21 and Modulates Gut Microbiota in COVID-19 Patients with Type 2 Diabetes, Viruses, doi:10.3390/v16020281.
Yang (D) et al., The effect of metformin on mortality and severity in COVID-19 patients with diabetes mellitus, Diabetes Research and Clinical Practice, doi:10.1016/j.diabres.2021.108977.
Lukito et al., The Effect of Metformin Consumption on Mortality in Hospitalized COVID-19 patients: a systematic review and meta-analysis, Diabetes & Metabolic Syndrome: Clinical Research & Reviews, doi:10.1016/j.dsx.2020.11.006.
Kow et al., Mortality risk with preadmission metformin use in patients with COVID-19 and diabetes: A meta-analysis, Journal of Medical Virology, doi:10.1002/jmv.26498.
Hariyanto (B) et al., Metformin use is associated with reduced mortality rate from coronavirus disease 2019 (COVID-19) infection, Obesity Medicine, doi:10.1016/j.obmed.2020.100290.
Ma (C) et al., Is metformin use associated with low mortality in patients with type 2 diabetes mellitus hospitalized for COVID-19? a multivariable and propensity score-adjusted meta-analysis, PLOS ONE, doi:10.1371/journal.pone.0282210.
Parveen et al., Association of Metformin with Mortality in COVID-19 Patients: A Systematic Review and Meta-Analysis, Annals of the National Academy of Medical Sciences (India), doi:10.1055/s-0042-1760353.
Li (I) et al., Metformin in Patients With COVID-19: A Systematic Review and Meta-Analysis, Frontiers in Medicine, doi:10.3389/fmed.2021.704666.
Schlesinger et al., Risk phenotypes of diabetes and association with COVID-19 severity and death: an update of a living systematic review and meta-analysis, Diabetologia, doi:10.1007/s00125-023-05928-1.
Petrelli et al., Metformin and Covid-19: a systematic review of systematic reviews with meta-analysis, Acta Biomedica Atenei Parmensis, doi:10.23750/abm.v94iS3.14405.
Oscanoa et al., Metformin therapy and severity and mortality of SARS-CoV-2 infection: a meta-analysis, Clinical Diabetology, doi:10.5603/DK.a2021.0035.
Kan et al., Mortality Risk of Antidiabetic Agents for Type 2 Diabetes With COVID-19: A Systematic Review and Meta-Analysis, Frontiers in Endocrinology, doi:10.3389/fendo.2021.708494.
Poly et al., Metformin Use Is Associated with Decreased Mortality in COVID-19 Patients with Diabetes: Evidence from Retrospective Studies and Biological Mechanism, Journal of Clinical Medicine, doi:10.3390/jcm10163507.
Song et al., The Effect of Antihyperglycemic Medications on COVID-19: A Meta-analysis and Systematic Review from Observational Studies, Therapeutic Innovation & Regulatory Science, doi:10.1007/s43441-024-00633-6.
Ganesh et al., Does metformin affect outcomes in COVID‐19 patients with new or pre‐existing diabetes mellitus? A systematic review and meta‐analysis, British Journal of Clinical Pharmacology, doi:10.1111/bcp.15258.
Nassar et al., Noninsulin‐based antihyperglycemic medications in patients with diabetes and COVID‐19: A systematic review and meta‐analysis, Journal of Diabetes, doi:10.1111/1753-0407.13359.
Zhan et al., Effect of Antidiabetic Therapy on Clinical Outcomes of COVID-19 Patients With Type 2 Diabetes: A Systematic Review and Meta-Analysis, Annals of Pharmacotherapy, doi:10.1177/10600280221133577.
Nguyen (B) et al., Preadmission use of antidiabetic medications and mortality among patients with COVID-19 having type 2 diabetes: A meta-analysis, Metabolism, doi:10.1016/j.metabol.2022.155196.
Han (C) et al., Association Between Anti-diabetic Agents and Clinical Outcomes of COVID-19 in Patients with Diabetes: A Systematic Review and Meta-Analysis, Archives of Medical Research, doi:10.1016/j.arcmed.2021.08.002.
Chen (J) et al., The Association Between Antidiabetic Agents and Clinical Outcomes of COVID-19 Patients With Diabetes: A Bayesian Network Meta-Analysis, Frontiers in Endocrinology, doi:10.3389/fendo.2022.895458.
Scheen, A., Metformin and COVID-19: From cellular mechanisms to reduced mortality, Diabetes & Metabolism, doi:10.1016/j.diabet.2020.07.006.
Sun (C) et al., Is Metformin Use Associated With a Decreased Mortality for COVID-19 Diabetic Patients? A Meta-Analysis, Journal of the Endocrine Society, doi:10.1210/jendso/bvab048.709.
Keels et al., Antidiabetic agent use and clinical outcomes in patients with diabetes hospitalized for COVID-19: a systematic review and meta-analysis, Frontiers in Endocrinology, doi:10.3389/fendo.2024.1482853.
Lee (B) et al., Metformin reduces the risk of developing influenza A virus related cardiovascular disease, Heliyon, doi:10.1016/j.heliyon.2023.e20284.
Kotani et al., Antiandrogen agents in COVID-19: a meta-analysis of randomized trials, Minerva Medica, doi:10.23736/S0026-4806.23.08538-5.
Cheema et al., Antiandrogens for the treatment of COVID‐19 patients: A meta‐analysis of randomized controlled trials, Journal of Medical Virology, doi:10.1002/jmv.28740.
Hamdan et al., In silico Evaluation of H1-Antihistamine as Potential Inhibitors of SARS-CoV-2 RNA-dependent RNA Polymerase: Repurposing Study of COVID-19 Therapy, Turkish Journal of Pharmaceutical Sciences, doi:10.4274/tjps.galenos.2024.49768.
Elshaier et al., Chlorpheniramine Maleate Displays Multiple Modes of Antiviral Action Against SARS-CoV-2: A Mechanistic Study, bioRxiv, doi:10.1101/2023.08.28.554806.
Black, S., Molecular Modeling and Preliminary Clinical Data Suggesting Antiviral Activity for Chlorpheniramine (Chlorphenamine) Against COVID-19, Cureus, doi:10.7759/cureus.20980.
Hou (B) et al., Testing of the inhibitory effects of loratadine and desloratadine on SARS-CoV-2 spike pseudotyped virus viropexis, Chemico-Biological Interactions, doi:10.1016/j.cbi.2021.109420.
Yu (E) et al., The histamine receptor H1 acts as an alternative receptor for SARS-CoV-2, mBio, doi:10.1128/mbio.01088-24.
Morin-Dewaele et al., Desloratadine, an FDA-approved cationic amphiphilic drug, inhibits SARS-CoV-2 infection in cell culture and primary human nasal epithelial cells by blocking viral entry, Scientific Reports, doi:10.1038/s41598-022-25399-5.
Rivas et al., Hydroxyzine inhibits SARS-CoV-2 Spike protein binding to ACE2 in a qualitative in vitro assay, bioRxiv, doi:10.1101/2021.01.04.424792.
Berkowitz et al., Sigma Receptor Ligands Prevent COVID Mortality In Vivo: Implications for Future Therapeutics, International Journal of Molecular Sciences, doi:10.3390/ijms242115718.
Al balawi et al., Assessing multi-target antiviral and antioxidant activities of natural compounds against SARS-CoV-2: an integrated in vitro and in silico study, Bioresources and Bioprocessing, doi:10.1186/s40643-024-00822-z.
Zhang (H) et al., Computational Discovery of Mitochondrial Dysfunction Biomarkers in Severe SARS-CoV-2 Infection: Facilitating Pytomedicine Screening, Phytomedicine, doi:10.1016/j.phymed.2024.155784.
Öztürkkan et al., In Silico investigation of the effects of curcuminoids on the spike protein of the omicron variant of SARS-CoV-2, Baku State University Journal of Chemistry and Material Sciences, 1:2, bsuj.bsu.edu.az/uploads/pdf/ec4204d62f7802de54e6092bf7860029.pdf.
Yunze et al., Therapeutic effect and potential mechanism of curcumin, an active ingredient in Tongnao Decoction, on COVID-19 combined with stroke: a network pharmacology study and GEO database mining, Research Square, doi:10.21203/rs.3.rs-4329762/v1.
Boseila et al., Throat spray formulated with virucidal Pharmaceutical excipients as an effective early prophylactic or treatment strategy against pharyngitis post-exposure to SARS CoV-2, European Journal of Pharmaceutics and Biopharmaceutics, doi:10.1016/j.ejpb.2024.114279.
Hidayah et al., Bioinformatics study of curcumin, demethoxycurcumin, bisdemethoxycurcumin and cyclocurcumin compounds in Curcuma longa as an antiviral agent via nucleocapsid on SARS-CoV-2 inhibition, International Conference on Organic and Applied Chemistry, doi:10.1063/5.0197724.
Singh (C) et al., Unlocking the potential of phytochemicals in inhibiting SARS-CoV-2 M Pro protein - An in-silico and cell-based approach, Research Square, doi:10.21203/rs.3.rs-3888947/v1.
Kant et al., Structure-based drug discovery to identify SARS-CoV2 spike protein–ACE2 interaction inhibitors, Journal of Biomolecular Structure and Dynamics, doi:10.1080/07391102.2023.2300060.
Naderi Beni et al., In silico studies of anti-oxidative and hot temperament-based phytochemicals as natural inhibitors of SARS-CoV-2 Mpro, PLOS ONE, doi:10.1371/journal.pone.0295014.
Moschovou et al., Exploring the Binding Effects of Natural Products and Antihypertensive Drugs on SARS-CoV-2: An In Silico Investigation of Main Protease and Spike Protein, International Journal of Molecular Sciences, doi:10.3390/ijms242115894.
Eleraky et al., Curcumin Transferosome-Loaded Thermosensitive Intranasal in situ Gel as Prospective Antiviral Therapy for SARS-Cov-2, International Journal of Nanomedicine, doi:10.2147/IJN.S423251.
Singh (D) et al., Computational studies to analyze effect of curcumin inhibition on coronavirus D614G mutated spike protein, The Seybold Report, doi:10.17605/OSF.IO/TKEXJ.
Thapa et al., In-silico Approach for Predicting the Inhibitory Effect of Home Remedies on Severe Acute Respiratory Syndrome Coronavirus-2, Makara Journal of Science, doi:10.7454/mss.v27i3.1609.
Srivastava et al., Paradigm of Well-Orchestrated Pharmacokinetic Properties of Curcuminoids Relative to Conventional Drugs for the Inactivation of SARS-CoV-2 Receptors: An In Silico Approach, Stresses, doi:10.3390/stresses3030043.
Winih Kinasih et al., Analisis in silico interaksi senyawa kurkuminoid terhadap enzim main protease 6LU7 dari SARS-CoV-2, Duta Pharma Journal, doi:10.47701/djp.v3i1.2904.
Wu (C) et al., Potential Mechanism of Curcumin and Resveratrol against SARS-CoV-2, Research Square, doi:10.21203/rs.3.rs-2780614/v1.
Nag et al., Curcumin inhibits spike protein of new SARS-CoV-2 variant of concern (VOC) Omicron, an in silico study, Computers in Biology and Medicine, doi:10.1016/j.compbiomed.2022.105552.
Rampogu et al., Molecular Docking and Molecular Dynamics Simulations Discover Curcumin Analogue as a Plausible Dual Inhibitor for SARS-CoV-2, International Journal of Molecular Sciences, doi:10.3390/ijms23031771.
Singh (E) et al., Potential of turmeric-derived compounds against RNA-dependent RNA polymerase of SARS-CoV-2: An in-silico approach, Computers in Biology and Medicine, doi:10.1016/j.compbiomed.2021.104965.
Kandeil et al., Bioactive Polyphenolic Compounds Showing Strong Antiviral Activities against Severe Acute Respiratory Syndrome Coronavirus 2, Pathogens, doi:10.3390/pathogens10060758.
Rajagopal et al., Activity of phytochemical constituents of Curcuma longa (turmeric) and Andrographis paniculata against coronavirus (COVID-19): an in silico approach, Future Journal of Pharmaceutical Sciences, doi:10.1186/s43094-020-00126-x.
Sekiou et al., In-Silico Identification of Potent Inhibitors of COVID-19 Main Protease (Mpro) and Angiotensin Converting Enzyme 2 (ACE2) from Natural Products: Quercetin, Hispidulin, and Cirsimaritin Exhibited Better Potential Inhibition than Hydroxy-Chloroquine Against COVID-19 Main Protease Active Site and ACE2, ChemRxiv, doi:10.26434/chemrxiv.12181404.v1.
Olubiyi et al., Novel dietary herbal preparations with inhibitory activities against multiple SARS-CoV-2 targets: A multidisciplinary investigation into antiviral activities, Food Chemistry Advances, doi:10.1016/j.focha.2025.100969.
Emam et al., Establishment of in-house assay for screening of anti-SARS-CoV-2 protein inhibitors, AMB Express, doi:10.1186/s13568-024-01739-8.
Van Tin et al., Spike Protein of SARS-CoV-2 Activates Cardiac Fibrogenesis through NLRP3 Inflammasomes and NF-κB Signaling, Cells, doi:10.3390/cells13161331.
Li (J) et al., Thermal shift assay (TSA)-based drug screening strategy for rapid discovery of inhibitors against the Nsp13 helicase of SARS-CoV-2, Animals and Zoonoses, doi:10.1016/j.azn.2024.06.001.
Kamble et al., Nanoparticulate curcumin spray imparts prophylactic and therapeutic properties against SARS-CoV-2, Emergent Materials, doi:10.1007/s42247-024-00754-6.
Nicoliche et al., Antiviral, anti-inflammatory and antioxidant effects of curcumin and curcuminoids in SH-SY5Y cells infected by SARS-CoV-2, Scientific Reports, doi:10.1038/s41598-024-61662-7.
Nittayananta et al., A novel film spray containing curcumin inhibits SARS-CoV-2 and influenza virus infection and enhances mucosal immunity, Virology Journal, doi:10.1186/s12985-023-02282-x.
Septisetyani et al., Curcumin and turmeric extract inhibited SARS-CoV-2 pseudovirus cell entry and Spike mediated cell fusion, bioRxiv, doi:10.1101/2023.09.28.560070.
Fam et al., Channel activity of SARS-CoV-2 viroporin ORF3a inhibited by adamantanes and phenolic plant metabolites, Scientific Reports, doi:10.1038/s41598-023-31764-9.
Teshima et al., Antiviral activity of curcumin and its analogs selected by an artificial intelligence-supported activity prediction system in SARS-CoV-2-infected VeroE6 cells, Natural Product Research, doi:10.1080/14786419.2023.2194647.
Leka et al., In vitro antiviral activity against SARS-CoV-2 of common herbal medicinal extracts and their bioactive compounds, Phytotherapy Research, doi:10.1002/ptr.7463.
Goc et al., Inhibitory effects of specific combination of natural compounds against SARS-CoV-2 and its Alpha, Beta, Gamma, Delta, Kappa, and Mu variants, European Journal of Microbiology and Immunology, doi:10.1556/1886.2021.00022.
Marín-Palma et al., Curcumin Inhibits In Vitro SARS-CoV-2 Infection In Vero E6 Cells through Multiple Antiviral Mechanisms, Molecules, doi:10.3390/molecules26226900.
Bahun et al., Inhibition of the SARS-CoV-2 3CLpro main protease by plant polyphenols, Food Chemistry, doi:10.1016/j.foodchem.2021.131594.
Bormann et al., Turmeric Root and Its Bioactive Ingredient Curcumin Effectively Neutralize SARS-CoV-2 In Vitro, Viruses, doi:10.3390/v13101914.
Guijarro-Real et al., Potential In Vitro Inhibition of Selected Plant Extracts against SARS-CoV-2 Chymotripsin-Like Protease (3CLPro) Activity, Foods, doi:10.3390/foods10071503.
Goc (B) et al., Phenolic compounds disrupt spike-mediated receptor-binding and entry of SARS-CoV-2 pseudo-virions, PLOS ONE, doi:10.1371/journal.pone.0253489.
Vahedian-Azimi et al., Effectiveness of Curcumin on Outcomes of Hospitalized COVID-19 Patients: A Systematic Review of Clinical Trials, Nutrients, doi:10.3390/nu14020256.
Kow (B) et al., The effect of curcumin on the risk of mortality in patients with COVID-19: A systematic review and meta-analysis of randomized trials, Phytotherapy Research, doi:10.1002/ptr.7468.
Shafiee et al., Curcumin for the treatment of COVID-19 patients: A meta-analysis of randomized controlled trials, Phytotherapy Research, doi:10.1002/ptr.7724.
Shojaei et al., The effectiveness of nano‐curcumin on patients with COVID‐19: A systematic review of clinical trials, Phytotherapy Research, doi:10.1002/ptr.7778.
Nelson et al., The Essential Medicinal Chemistry of Curcumin, Journal of Medicinal Chemistry, doi:10.1021/acs.jmedchem.6b00975.
Hegde et al., Curcumin Formulations for Better Bioavailability: What We Learned from Clinical Trials Thus Far?, ACS Omega, doi:10.1021/acsomega.2c07326.
Acharya et al., Potential therapeutic intervention of melatonin against COVID-19: A comparative pharmacokinetic study, Melatonin Research, doi:10.32794/mr112500181.
Kumar Yadalam et al., Assessing the therapeutic potential of angomelatine, ramelteon, and melatonin against SARS-Cov-2, Saudi Journal of Biological Sciences, doi:10.1016/j.sjbs.2022.01.049.
Fernandes et al., Melatonin-Index as a biomarker for predicting the distribution of presymptomatic and asymptomatic SARS-CoV-2 carriers, Melatonin Research, doi:10.32794/mr11250090.
Šeškutė et al., Antiviral Effect of Melatonin on Caco-2 Cell Organoid Culture: Trick or Treat?, International Journal of Molecular Sciences, doi:10.3390/ijms252211872.
Cecon et al., Melatonin drugs inhibit SARS-CoV-2 entry into the brain and virus-induced damage of cerebral small vessels, bioRxiv, doi:10.1101/2021.12.30.474561.
Cecon (B) et al., Therapeutic potential of melatonin and melatonergic drugs on K18-hACE2 mice infected with SARS-CoV-2, Journal of Pineal Research, doi:10.1111/jpi.12772.
Soto (C) et al., Redox Homeostasis Alteration Is Restored through Melatonin Treatment in COVID-19 Patients: A Preliminary Study, International Journal of Molecular Sciences, doi:10.3390/ijms25084543.
Xie et al., The role of reactive oxygen species in severe acute respiratory syndrome coronavirus 2 (SARS-COV-2) infection-induced cell death, Cellular & Molecular Biology Letters, doi:10.1186/s11658-024-00659-6.
Lan et al., Efficacy of Melatonin in the Treatment of Patients With COVID-19: A Systematic Review and Meta-Analysis of Randomized Controlled Trials, Journal of Medical Virology, doi:10.1002/jmv.27595.
Pilia et al., Does melatonin reduce mortality in COVID-19?, Annals of Medicine and Surgery, doi:10.1016/j.amsu.2022.103817.
Wang (H) et al., The safety and efficacy of melatonin in the treatment of COVID-19: A systematic review and meta-analysis, Medicine, doi:10.1097/MD.0000000000030874.
Tóth et al., Melatonin as adjuvant treatment in COVID-19 patients. A meta-analysis of randomized and propensity matched studies, Signa Vitae, doi:10.22514/sv.2023.076.
Taha et al., Safety and efficacy of melatonin as an adjuvant therapy in COVID-19 patients: Systematic review and meta-analysis, Advances in Medical Sciences, doi:10.1016/j.advms.2023.09.007.
Amin et al., Role of Melatonin in Management of COVID-19: A Systematic Review, Microbes, Infection and Chemotherapy, doi:10.54034/mic.e1982.
Qin (B) et al., Benefits of melatonin on mortality in severe-to-critical COVID-19 patients: A systematic review and meta-analysis of randomized controlled trials, Clinics, doi:10.1016/j.clinsp.2025.100638.
Zein (B) et al., Effect of colchicine on mortality in patients with COVID-19 – A systematic review and meta-analysis, Diabetes & Metabolic Syndrome: Clinical Research & Reviews, doi:10.1016/j.dsx.2022.102395.
Yasmin et al., Safety and efficacy of colchicine in COVID-19 patients: A systematic review and meta-analysis of randomized control trials, PLOS ONE, doi:10.1371/journal.pone.0266245.
Rai et al., The Potential Role of Colchicine in Reducing Mortality and Mechanical Ventilation Rates in COVID-19 Infection: A Meta-analysis, Journal of Advances in Medicine and Medical Research, doi:10.9734/jammr/2022/v34i2031503.
Elshafei et al., Colchicine use might be associated with lower mortality in COVID‐19 patients: A meta‐analysis, European Journal of Clinical Investigation, doi:10.1111/eci.13645.
Lien et al., Repurposing Colchicine in Treating Patients with COVID-19: A Systematic Review and Meta-Analysis, Life, doi:10.3390/life11080864.
Danjuma et al., Does Colchicine Reduce Mortality in Patients with Covid-19 Clinical Syndrome? An Umbrella Review of Published Meta-Analyses, Elsevier BV, doi:10.2139/ssrn.4447127.
Salah et al., Meta-analysis of the Effect of Colchicine on Mortality and Mechanical Ventilation in COVID-19, The American Journal of Cardiology, doi:10.1016/j.amjcard.2021.02.005.
Golpour et al., The effectiveness of Colchicine as an anti-inflammatory drug in the treatment of coronavirus disease 2019: Meta-analysis, International Journal of Immunopathology and Pharmacology, doi:10.1177/20587384211031763.
Elshiwy et al., The role of colchicine in the management of COVID-19: a meta-analysis, BMC Pulmonary Medicine, doi:10.1186/s12890-024-03001-0.
Kow (C) et al., The effect of colchicine on mortality outcome and duration of hospital stay in patients with COVID‐19: A meta‐analysis of randomized trials, Immunity, Inflammation and Disease, doi:10.1002/iid3.562.
Neris Almeida Viana et al., Benefits of probiotic use on COVID-19: A systematic review and meta-analysis, Critical Reviews in Food Science and Nutrition, doi:10.1080/10408398.2022.2128713.
Tian et al., Probiotics improve symptoms of patients with COVID-19 through gut-lung axis: a systematic review and meta-analysis, Frontiers in Nutrition, doi:10.3389/fnut.2023.1179432.
Kobatake et al., Lactobacillus paragasseri SBT2055 Activates Plasmacytoid Dendritic Cells and Improves Subjective Symptoms of Common Cold in Healthy Adults: A Randomized, Double-Blind, Placebo-Controlled Parallel-Group Comparative Trial, Nutrients, doi:10.3390/nu15204458.
Reis (F) et al., Antiviral effect of Bromelain combined with acetylcysteine against SARS-CoV-2 Omicron variant, Scientific Reports, doi:10.1038/s41598-025-92242-y.
Chaopreecha et al., Andrographolide attenuates SARS-CoV-2 infection via an up-regulation of glutamate-cysteine ligase catalytic subunit (GCLC), Phytomedicine, doi:10.1016/j.phymed.2024.156279.
Frasson et al., Identification of druggable host dependency factors shared by multiple SARS-CoV-2 variants of concern, Journal of Molecular Cell Biology. doi:10.1093/jmcb/mjae004, academic.oup.com/jmcb/advance-article/doi/10.1093/jmcb/mjae004/7596546.
Ferreira (D) et al., Taming the SARS-CoV-2-mediated proinflammatory response with BromAc®, Frontiers in Immunology, doi:10.3389/fimmu.2023.1308477.
La Maestra et al., Inhibition of the Cell Uptake of Delta and Omicron SARS-CoV-2 Pseudoviruses by N-Acetylcysteine Irrespective of the Oxidoreductive Environment, Cells, doi:10.3390/cells11203313.
Goc (C) et al., Inhibitory effects of specific combination of natural compounds against SARS-CoV-2 and its Alpha, Beta, Gamma, Delta, Kappa, and Mu variants, European Journal of Microbiology and Immunology, doi:10.1556/1886.2021.00022.
Akhter et al., The Combination of Bromelain and Acetylcysteine (BromAc) Synergistically Inactivates SARS-CoV-2, Viruses, doi:10.3390/v13030425.
Yuan (C) et al., The role of cell death in SARS-CoV-2 infection, Signal Transduction and Targeted Therapy, doi:10.1038/s41392-023-01580-8.
Alam (B) et al., N-acetylcysteine reduces severity and mortality in COVID-19 patients: A systematic review and meta-analysis, Journal of Advanced Veterinary and Animal Research, doi:10.5455/javar.2023.j665.
De Flora et al., Attenuation of influenza-like symptomatology and improvement of cell-mediated immunity with long-term N-acetylcysteine treatment, European Respiratory Journal, doi:10.1183/09031936.97.10071535.
Alkafaas (B) et al., Molecular docking as a tool for the discovery of novel insight about the role of acid sphingomyelinase inhibitors in SARS- CoV-2 infectivity, BMC Public Health, doi:10.1186/s12889-024-17747-z.
Abatematteo et al., A conformational rearrangement of the SARS-CoV-2 host protein sigma-1 is required for antiviral activity: insights from a combined in-silico/in-vitro approach, Scientific Reports, doi:10.1038/s41598-023-39662-w.
Zhang (I) et al., SARS-CoV-2 NSP6 reduces autophagosome size and affects viral replication via sigma-1 receptor, Journal of Virology, doi:10.1128/jvi.00754-24.
Lee (C) et al., Fluvoxamine for Outpatient Management of COVID-19 to Prevent Hospitalization: A Systematic Review and Meta-analysis, JAMA Network Open, doi:10.1001/jamanetworkopen.2022.6269.
Nakhaee et al., The effect of antidepressants on the severity of COVID-19 in hospitalized patients: A systematic review and meta-analysis, PLOS ONE, doi:10.1371/journal.pone.0267423.
Lu et al., Effect of fluvoxamine on outcomes of nonhospitalized patients with COVID-19: A systematic review and meta-analysis, Journal of Infection and Public Health, doi:10.1016/j.jiph.2022.10.010.
Marcec et al., A meta-analysis regarding fluvoxamine and hospitalization risk of COVID-19 patients: TOGETHER making a difference, Journal of Infection, doi:10.1016/j.jinf.2022.11.011.
Deng et al., Efficacy and safety of selective serotonin reuptake inhibitors in COVID-19 management: A systematic review and meta-analysis, Clinical Microbiology and Infection, doi:10.1016/j.cmi.2023.01.010.
Deng (B) et al., Evaluating fluvoxamine for the outpatient treatment of COVID‐19: A systematic review and meta‐analysis, Reviews in Medical Virology, doi:10.1002/rmv.2501.
Zhou (E) et al., The efficacy and safety of fluvoxamine in patients with COVID-19: A systematic review and meta-analysis from randomized controlled trials, PLOS ONE, doi:10.1371/journal.pone.0300512.
Prasanth et al., A systematic review and meta-analysis, investigating dose and time of fluvoxamine treatment efficacy for COVID-19 clinical deterioration, death, and Long-COVID complications, Scientific Reports, doi:10.1038/s41598-024-64260-9.
Li (K) et al., Azvudine alleviates SARS-CoV-2-induced inflammation by targeting myeloperoxidase in NETosis, Chinese Chemical Letters, doi:10.1016/j.cclet.2024.110238.
Zheng (B) et al., Small-molecule antivirals treatment for COVID-19: A systematic review and network meta-analysis, International Journal of Antimicrobial Agents, doi:10.1016/j.ijantimicag.2024.107096.
Wang (I) et al., Effectiveness of azvudine in reducing mortality of COVID-19 patients: a systematic review and meta-analysis, Virology Journal, doi:10.1186/s12985-024-02316-y.
Amani et al., Effectiveness and safety of azvudine in COVID-19: A systematic review and meta-analysis, PLOS ONE, doi:10.1371/journal.pone.0298772.
Dong et al., Efficacy and Safety of Azvudine in Patients With COVID‐19 in China: A Meta‐Analysis of Observational Studies, The Clinical Respiratory Journal, doi:10.1111/crj.13798.
Rahman (B) et al., In silico Screening of Potential Drug Candidate against Chain a of Coronavirus Binding Protein from Major Nigella Bioactive Compounds, Asian Journal of Advanced Research and Reports, doi:10.9734/ajarr/2024/v18i7697.
Zafar Nayak Snehasis, S., Molecular Docking to Discover Potential Bio-Extract Substitutes for Hydroxychloroquine against COVID-19 and Malaria, International Journal of Science and Research (IJSR), doi:10.21275/SR24323192940.
Ali et al., Computational Prediction of Nigella sativa Compounds as Potential Drug Agents for Targeting Spike Protein of SARS-CoV-2, Pakistan BioMedical Journal, doi:10.54393/pbmj.v6i3.853.
Miraz et al., Nigelladine A among Selected Compounds from Nigella sativa Exhibits Propitious Interaction with Omicron Variant of SARS-CoV-2: An In Silico Study, International Journal of Clinical Practice, doi:10.1155/2023/9917306.
Sherwani et al., Pharmacological Profile of Nigella sativa Seeds in Combating COVID-19 through In-Vitro and Molecular Docking Studies, Processes, doi:10.3390/pr10071346.
Khan (D) et al., Inhibitory effect of thymoquinone from Nigella sativa against SARS-CoV-2 main protease. An in-silico study, Brazilian Journal of Biology, doi:10.1590/1519-6984.25066.
Esharkawy et al., In vitro Potential Antiviral SARS-CoV-19- Activity of Natural Product Thymohydroquinone and Dithymoquinone from Nigela sativia, Bioorganic Chemistry, doi:10.1016/j.bioorg.2021.105587.
Banerjee et al., Nigellidine (Nigella sativa, black-cumin seed) docking to SARS CoV-2 nsp3 and host inflammatory proteins may inhibit viral replication/transcription, Natural Product Research, doi:10.1080/14786419.2021.2018430.
Rizvi et al., Identifying the Most Potent Dual-Targeting Compound(s) against 3CLprotease and NSP15exonuclease of SARS-CoV-2 from Nigella sativa: Virtual Screening via Physicochemical Properties, Docking and Dynamic Simulation Analysis, Processes, doi:10.3390/pr9101814.
Mir et al., Identification of SARS-CoV-2 RNA-dependent RNA polymerase inhibitors from the major phytochemicals of Nigella sativa: An in silico approach, Saudi Journal of Biological Sciences, doi:10.1016/j.sjbs.2021.09.002.
Hardianto et al., Exploring the Potency of Nigella sativa Seed in Inhibiting SARS-CoV-2 Main Protease Using Molecular Docking and Molecular Dynamics Simulations, Indonesian Journal of Chemistry, doi:10.22146/ijc.65951.
Maiti et al., Active-site Molecular docking of Nigellidine to nucleocapsid/Nsp2/Nsp3/MPro of COVID-19 and to human IL1R and TNFR1/2 may stop viral-growth/cytokine-flood, and the drug source Nigella sativa (black cumin) seeds show potent antioxidant role in experimental rats, Research Square, doi:10.21203/rs.3.rs-26464/v1.
Duru et al., In silico identification of compounds from Nigella sativa seed oil as potential inhibitors of SARS-CoV-2 targets, Bulletin of the National Research Centre, doi:10.1186/s42269-021-00517-x.
Bouchentouf et al., Identification of Compounds from Nigella Sativa as New Potential Inhibitors of 2019 Novel Coronasvirus (Covid-19): Molecular Docking Study, ChemRxiv, doi:10.26434/chemrxiv.12055716.v1.
Ali (B) et al., In vitro inhibitory effect of Nigella sativa L. extracts on SARS-COV-2 spike protein-ACE2 interaction, Current Therapeutic Research, doi:10.1016/j.curtheres.2024.100759.
Bostancıklıoğlu et al., Nigella sativa, Anthemis hyaline and Citrus sinensis extracts reduce SARS-CoV-2 replication by fluctuating Rho GTPase, PI3K-AKT, and MAPK/ERK pathways in HeLa-CEACAM1a cells, Gene, doi:10.1016/j.gene.2024.148366.
Maen et al., Individual ingredients of NP-101 (Thymoquinone formula) inhibit SARS-CoV-2 pseudovirus infection, Frontiers in Pharmacology, doi:10.3389/fphar.2024.1291212.
Saghghaei et al., Protective effects of Nigella sativa oil thymoquinone and dexamethasone on bleomycin-induced lung fibrosis in rats, Veterinary Research Forum, doi:10.30466/vrf.2024.2024154.4196.
Kow (D) et al., The effect of Nigella sativa on the risk of mortality in patients with COVID‐19: A systematic review and meta‐analysis of randomized trials, Phytotherapy Research, doi:10.1002/ptr.7743.
Umer et al., Nigella sativa for the treatment of COVID-19 patients: A rapid systematic review and meta-analysis of randomized controlled trials, Food Science & Nutrition, doi:10.1002/fsn3.3906.
Makoana et al., Integration of metabolomics and chemometrics with in-silico and in-vitro approaches to unravel SARS-Cov-2 inhibitors from South African plants, PLOS ONE, doi:10.1371/journal.pone.0320415.
Moharram et al., Secondary metabolites of Alternaria alternate appraisal of their SARS-CoV-2 inhibitory and anti-inflammatory potentials, PLOS ONE, doi:10.1371/journal.pone.0313616.
Metwaly et al., Integrated study of Quercetin as a potent SARS-CoV-2 RdRp inhibitor: Binding interactions, MD simulations, and In vitro assays, PLOS ONE, doi:10.1371/journal.pone.0312866.
Pan et al., Decoding the mechanism of Qingjie formula in the prevention of COVID-19 based on network pharmacology and molecular docking, Heliyon, doi:10.1016/j.heliyon.2024.e39167.
Xu (C) et al., Quercetin inhibited LPS-induced cytokine storm by interacting with the AKT1-FoxO1 and Keap1-Nrf2 signaling pathway in macrophages, Scientific Reports, doi:10.1038/s41598-024-71569-y.
Tamil Selvan et al., Computational Investigations to Identify Potent Natural Flavonoid Inhibitors of the Nonstructural Protein (NSP) 16/10 Complex Against Coronavirus, Cureus, doi:10.7759/cureus.68098.
Sunita et al., Characterization of Phytochemical Inhibitors of the COVID-19 Primary Protease Using Molecular Modelling Approach, Asian Journal of Microbiology and Biotechnology, doi:10.56557/ajmab/2024/v9i28800.
Wu (D) et al., Biomarkers Prediction and Immune Landscape in Covid-19 and “Brain Fog”, Elsevier BV, doi:10.2139/ssrn.4897774.
Raman et al., Phytoconstituents of Citrus limon (Lemon) as Potential Inhibitors Against Multi Targets of SARS‐CoV‐2 by Use of Molecular Modelling and In Vitro Determination Approaches, ChemistryOpen, doi:10.1002/open.202300198.
Asad et al., Exploring the antiviral activity of Adhatoda beddomei bioactive compounds in interaction with coronavirus spike protein, Archives of Medical Reports, 1:1, archmedrep.com/index.php/amr/article/view/3.
Irfan et al., Phytoconstituents of Artemisia Annua as potential inhibitors of SARS CoV2 main protease: an in silico study, BMC Infectious Diseases, doi:10.1186/s12879-024-09387-w.
Yuan (D) et al., Network pharmacology and molecular docking reveal the mechanisms of action of Panax notoginseng against post-COVID-19 thromboembolism, Review of Clinical Pharmacology and Pharmacokinetics - International Edition, doi:10.61873/DTFA3974.
Nalban et al., Targeting COVID-19 (SARS-CoV-2) main protease through phytochemicals of Albizia lebbeck: molecular docking, molecular dynamics simulation, MM–PBSA free energy calculations, and DFT analysis, Journal of Proteins and Proteomics, doi:10.1007/s42485-024-00136-w.
Zhou (F) et al., Bioinformatics and system biology approaches to determine the connection of SARS-CoV-2 infection and intrahepatic cholangiocarcinoma, PLOS ONE, doi:10.1371/journal.pone.0300441.
Waqas et al., Discovery of Novel Natural Inhibitors Against SARS-CoV-2 Main Protease: A Rational Approach to Antiviral Therapeutics, Current Medicinal Chemistry, doi:10.2174/0109298673292839240329081008.
Hasanah et al., Decoding the therapeutic potential of empon-empon: a bioinformatics expedition unraveling mechanisms against COVID-19 and atherosclerosis, International Journal of Applied Pharmaceutics, doi:10.22159/ijap.2024v16i2.50128.
Shaik et al., Computational identification of selected bioactive compounds from Cedrus deodara as inhibitors against SARS-CoV-2 main protease: a pharmacoinformatics study, Indian Drugs, doi:10.53879/id.61.02.13859.
Singh (F) et al., Unlocking the potential of phytochemicals in inhibiting SARS-CoV-2 M Pro protein - An in-silico and cell-based approach, Research Square, doi:10.21203/rs.3.rs-3888947/v1.
El-Megharbel et al., Chemical and spectroscopic characterization of (Artemisinin/Quercetin/ Zinc) novel mixed ligand complex with assessment of its potent high antiviral activity against SARS-CoV-2 and antioxidant capacity against toxicity induced by acrylamide in male rats, PeerJ, doi:10.7717/peerj.15638.
Akinwumi et al., Evaluation of therapeutic potentials of some bioactive compounds in selected African plants targeting main protease (Mpro) in SARS-CoV-2: a molecular docking study, Egyptian Journal of Medical Human Genetics, doi:10.1186/s43042-023-00456-4.
Yang (E) et al., Active ingredient and mechanistic analysis of traditional Chinese medicine formulas for the prevention and treatment of COVID-19: Insights from bioinformatics and in vitro experiments, Medicine, doi:10.1097/MD.0000000000036238.
Chandran et al., Molecular docking analysis of quercetin with known CoVid-19 targets, Bioinformation, doi:10.6026/973206300191081.
Qin (C) et al., Exploring the bioactive compounds of Feiduqing formula for the prevention and management of COVID-19 through network pharmacology and molecular docking, Medical Data Mining, doi:10.53388/MDM202407003.
Pan (B) et al., Quercetin: A promising drug candidate against the potential SARS-CoV-2-Spike mutants with high viral infectivity, Computational and Structural Biotechnology Journal, doi:10.1016/j.csbj.2023.10.029.
Ahmed (C) et al., Evaluation of the Effect of Zinc, Quercetin, Bromelain and Vitamin C on COVID-19 Patients, International Journal of Diabetes Management, doi:10.61797/ijdm.v2i2.259.
Singh (G) et al., Flavonoids as Potent Inhibitor of SARS-CoV-2 Nsp13 Helicase: Grid Based Docking Approach, Middle East Research Journal of Pharmaceutical Sciences, doi:10.36348/merjps.2023.v03i04.001.
Mandal et al., In silico anti-viral assessment of phytoconstituents in a traditional (Siddha Medicine) polyherbal formulation – Targeting Mpro and pan-coronavirus post-fusion Spike protein, Journal of Traditional and Complementary Medicine, doi:10.1016/j.jtcme.2023.07.004.
Sai Ramesh et al., Computational analysis of the phytocompounds of Mimusops elengi against spike protein of SARS CoV2 – An Insilico model, International Journal of Biological Macromolecules, doi:10.1016/j.ijbiomac.2023.125553.
Corbo et al., Inhibitory potential of phytochemicals on five SARS-CoV-2 proteins: in silico evaluation of endemic plants of Bosnia and Herzegovina, Biotechnology & Biotechnological Equipment, doi:10.1080/13102818.2023.2222196.
Azmi et al., Utilization of quercetin flavonoid compounds in onion (Allium cepa L.) as an inhibitor of SARS-CoV-2 spike protein against ACE2 receptors, 11th International Seminar on New Paradigm and Innovation on Natural Sciences and its Application, doi:10.1063/5.0140285.
Alanzi et al., Structure-based virtual identification of natural inhibitors of SARS-CoV-2 and its Delta and Omicron variant proteins, Future Virology, doi:10.2217/fvl-2022-0184.
Yang (F) et al., In silico evidence implicating novel mechanisms of Prunella vulgaris L. as a potential botanical drug against COVID-19-associated acute kidney injury, Frontiers in Pharmacology, doi:10.3389/fphar.2023.1188086.
Wang (J) et al., Computational Analysis of Lianhua Qingwen as an Adjuvant Treatment in Patients with COVID-19, Society of Toxicology Conference, 2023, www.researchgate.net/publication/370491709_Y_Wang_A_E_Tan_O_Chew_A_Hsueh_and_D_E_Johnson_2023_Computational_Analysis_of_Lianhua_Qingwen_as_an_Adjuvant_Treatment_in_Patients_with_COVID-19_Toxicologist_1921_507.
Ibeh et al., Computational studies of potential antiviral compounds from some selected Nigerian medicinal plants against SARS-CoV-2 proteins, Informatics in Medicine Unlocked, doi:10.1016/j.imu.2023.101230.
Alavi et al., Interaction of Epigallocatechin Gallate and Quercetin with Spike Glycoprotein (S-Glycoprotein) of SARS-CoV-2: In Silico Study, Biomedicines, doi:10.3390/biomedicines10123074.
Chellasamy (B) et al., Docking and molecular dynamics studies of human ezrin protein with a modelled SARS-CoV-2 endodomain and their interaction with potential invasion inhibitors, Journal of King Saud University - Science, doi:10.1016/j.jksus.2022.102277.
Şimşek et al., In silico identification of SARS-CoV-2 cell entry inhibitors from selected natural antivirals, Journal of Molecular Graphics and Modelling, doi:10.1016/j.jmgm.2021.108038.
Kandeil (B) et al., Bioactive Polyphenolic Compounds Showing Strong Antiviral Activities against Severe Acute Respiratory Syndrome Coronavirus 2, Pathogens, doi:10.3390/pathogens10060758.
Rehman et al., Natural Compounds as Inhibitors of SARS-CoV-2 Main Protease (3CLpro): A Molecular Docking and Simulation Approach to Combat COVID-19, Current Pharmaceutical Design, doi:10.2174/1381612826999201116195851.
Zhang (J) et al., In silico screening of Chinese herbal medicines with the potential to directly inhibit 2019 novel coronavirus, Journal of Integrative Medicine, doi:10.1016/j.joim.2020.02.005.
Spinelli et al., Amphibian‐Derived Peptides as Natural Inhibitors of SARS‐CoV‐2 Main Protease (Mpro): A Combined In Vitro and In Silico Approach, Chemistry & Biodiversity, doi:10.1002/cbdv.202403202.
Aguilera-Rodriguez et al., Inhibition of SARS-CoV-2 3CLpro by chemically modified tyrosinase from Agaricus bisporus, RSC Medicinal Chemistry, doi:10.1039/D4MD00289J.
Fang et al., Development of nanoparticles incorporated with quercetin and ACE2-membrane as a novel therapy for COVID-19, Journal of Nanobiotechnology, doi:10.1186/s12951-024-02435-2.
Roy (C) et al., Quercetin inhibits SARS-CoV-2 infection and prevents syncytium formation by cells co-expressing the viral spike protein and human ACE2, Virology Journal, doi:10.1186/s12985-024-02299-w.
DiGuilio et al., Quercetin improves and protects Calu-3 airway epithelial barrier function, Frontiers in Cell and Developmental Biology, doi:10.3389/fcell.2023.1271201.
Zhang (K) et al., Discovery of the covalent SARS‐CoV‐2 Mpro inhibitors from antiviral herbs via integrating target‐based high‐throughput screening and chemoproteomic approaches, Journal of Medical Virology, doi:10.1002/jmv.29208.
Wu (E) et al., SARS-CoV-2 N protein induced acute kidney injury in diabetic db/db mice is associated with a Mincle-dependent M1 macrophage activation, Frontiers in Immunology, doi:10.3389/fimmu.2023.1264447.
Xu (D) et al., Bioactive compounds from Huashi Baidu decoction possess both antiviral and anti-inflammatory effects against COVID-19, Proceedings of the National Academy of Sciences, doi:10.1073/pnas.2301775120.
Aguado et al., Senolytic therapy alleviates physiological human brain aging and COVID-19 neuropathology, bioRxiv, doi:10.1101/2023.01.17.524329.
Goc (D) et al., Inhibitory effects of specific combination of natural compounds against SARS-CoV-2 and its Alpha, Beta, Gamma, Delta, Kappa, and Mu variants, European Journal of Microbiology and Immunology, doi:10.1556/1886.2021.00022.
Munafò et al., Quercetin and Luteolin Are Single-digit Micromolar Inhibitors of the SARS-CoV-2 RNA-dependent RNA Polymerase, Research Square, doi:10.21203/rs.3.rs-1149846/v1.
Singh (H) et al., The spike protein of SARS-CoV-2 virus induces heme oxygenase-1: Pathophysiologic implications, Biochimica et Biophysica Acta (BBA) - Molecular Basis of Disease, doi:10.1016/j.bbadis.2021.166322.
Shaker et al., Anti-cytokine Storm Activity of Fraxin, Quercetin, and their Combination on Lipopolysaccharide-Induced Cytokine Storm in Mice: Implications in COVID-19, Iranian Journal of Medical Sciences, doi:10.30476/ijms.2023.98947.3102.
Wu (F) et al., Treatment with Quercetin inhibits SARS-CoV-2 N protein-induced acute kidney injury by blocking Smad3-dependent G1 cell cycle arrest, Molecular Therapy, doi:10.1016/j.ymthe.2022.12.002.
Azmi (B) et al., The role of vitamin D receptor and IL‐6 in COVID‐19, Molecular Genetics & Genomic Medicine, doi:10.1002/mgg3.2172.
Cheema (B) et al., Quercetin for the treatment of COVID-19 patients: A systematic review and meta-analysis, Reviews in Medical Virology, doi:10.1002/rmv.2427.
Ziaei et al., The effect of quercetin supplementation on clinical outcomes in COVID‐19 patients: A systematic review and meta‐analysis, Food Science & Nutrition, doi:10.1002/fsn3.3715.
Ostrov et al., Highly Specific Sigma Receptor Ligands Exhibit Anti-Viral Properties in SARS-CoV-2 Infected Cells, Pathogens, doi:10.3390/pathogens10111514.
Alsaidi et al., Griffithsin and Carrageenan Combination Results in Antiviral Synergy against SARS-CoV-1 and 2 in a Pseudoviral Model, Marine Drugs, doi:10.3390/md19080418.
Wan et al., Synergistic inhibition effects of andrographolide and baicalin on coronavirus mechanisms by downregulation of ACE2 protein level, Scientific Reports, doi:10.1038/s41598-024-54722-5.
Said (C) et al., The effect of Nigella sativa and vitamin D3 supplementation on the clinical outcome in COVID-19 patients: A randomized controlled clinical trial, Frontiers in Pharmacology, doi:10.3389/fphar.2022.1011522.
Fiaschi et al., In Vitro Combinatorial Activity of Direct Acting Antivirals and Monoclonal Antibodies against the Ancestral B.1 and BQ.1.1 SARS-CoV-2 Viral Variants, Viruses, doi:10.3390/v16020168.
Xing et al., Published anti-SARS-CoV-2 in vitro hits share common mechanisms of action that synergize with antivirals, Briefings in Bioinformatics, doi:10.1093/bib/bbab249.
Chen (K) et al., Synergistic Inhibition of SARS-CoV-2 Replication Using Disulfiram/Ebselen and Remdesivir, ACS Pharmacology & Translational Science, doi:10.1021/acsptsci.1c00022.
Hempel et al., Synergistic inhibition of SARS-CoV-2 cell entry by otamixaban and covalent protease inhibitors: pre-clinical assessment of pharmacological and molecular properties, Chemical Science, doi:10.1039/D1SC01494C.
Schultz et al., Pyrimidine inhibitors synergize with nucleoside analogues to block SARS-CoV-2, Nature, doi:10.1038/s41586-022-04482-x.
Ohashi et al., Potential anti-COVID-19 agents, cepharanthine and nelfinavir, and their usage for combination treatment, iScience, doi:10.1016/j.isci.2021.102367.
Al Krad et al., The protease inhibitor Nirmatrelvir synergizes with inhibitors of GRP78 to suppress SARS-CoV-2 replication, bioRxiv, doi:10.1101/2025.03.09.642200.
Zhou (G) et al., Nirmatrelvir-resistant SARS-CoV-2 variants with high fitness in an infectious cell culture system, Science Advances, doi:10.1126/sciadv.add7197.
Jochmans et al., The Substitutions L50F, E166A, and L167F in SARS-CoV-2 3CLpro Are Selected by a Protease Inhibitor In Vitro and Confer Resistance To Nirmatrelvir, mBio, doi:10.1128/mbio.02815-22.
Lopez et al., SARS-CoV-2 Resistance to Small Molecule Inhibitors, Current Clinical Microbiology Reports, doi:10.1007/s40588-024-00229-6.
Zvornicanin et al., Molecular Mechanisms of Drug Resistance and Compensation in SARS-CoV-2 Main Protease: The Interplay Between E166 and L50, bioRxiv, doi:10.1101/2025.01.24.634813.
Vukovikj et al., Impact of SARS-CoV-2 variant mutations on susceptibility to monoclonal antibodies and antiviral drugs: a non-systematic review, April 2022 to October 2024, Eurosurveillance, doi:10.2807/1560-7917.ES.2025.30.10.2400252.
Iriyama et al., Clinical and molecular landscape of prolonged SARS-CoV-2 infection with resistance to remdesivir in immunocompromised patients, PNAS Nexus, doi:10.1093/pnasnexus/pgaf085.
Moghadasi et al., Rapid resistance profiling of SARS-CoV-2 protease inhibitors, npj Antimicrobials and Resistance, doi:10.1038/s44259-023-00009-0.
Zhang (L) et al., Virological Traits of the SARS-CoV-2 BA.2.87.1 Lineage, Vaccines, doi:10.3390/vaccines12050487.
Rudraraju et al., Parallel use of human stem cell lung and heart models provide insights for SARS-CoV-2 treatment, Stem Cell Reports, doi:10.1016/j.stemcr.2023.05.007.
Wojciulik et al., The impact of genetic polymorphism on course and severity of the SARS-CoV-2 infection and COVID-19 disease, Przeglad Epidemiologiczny, doi:10.32394/pe/194862.
Yip (B) et al., The role of inflammatory gene polymorphisms in severe COVID-19: a review, Virology Journal, doi:10.1186/s12985-024-02597-3.
Serra-Llovich et al., Pharmacogenomic Study of SARS-CoV-2 Treatments: Identifying Polymorphisms Associated with Treatment Response in COVID-19 Patients, Biomedicines, doi:10.3390/biomedicines13030553.
İdikut et al., Association of Endothelial Nitric Oxide Synthase Polymorphisms with Clinical Severity in Patients with COVID-19, Journal of Clinical Medicine, doi:10.3390/jcm14061931.
Please send us corrections, updates, or comments.
c19early involves the extraction of 100,000+ datapoints from
thousands of papers. Community updates
help ensure high accuracy.
Treatments and other interventions are complementary.
All practical, effective, and safe
means should be used based on risk/benefit analysis.
No treatment or intervention is 100% available and effective for all current
and future variants.
We do not provide medical advice. Before taking any medication,
consult a qualified physician who can provide personalized advice and details
of risks and benefits based on your medical history and situation. IMA and WCH
provide treatment protocols.
Thanks for your feedback! Please search before submitting papers and note
that studies are listed under the date they were first available, which may be
the date of an earlier preprint.